Nucleic acids are the fundamental molecules of life, serving as the blueprint for all living organisms. These complex macromolecules store and transmit genetic information, orchestrating the development, functioning, and reproduction of all known living things. In this comprehensive guide, we will delve deep into the components of nucleic acids, exploring their structure, types, functions, and significance in modern biology and biotechnology.
As we embark on this journey through the world of nucleic acids, we’ll unravel the intricate details of their building blocks, examine the differences between DNA and RNA, and discover how these molecules shape life as we know it. Whether you’re a student, researcher, or simply curious about the molecular basis of life, this article will provide you with a thorough understanding of nucleic acids and their crucial role in biology.
What are Nucleic Acids?
Nucleic acids are large, complex biomolecules that play essential roles in all known forms of life. They are polymers made up of nucleotides, which are repeating units bound together by covalent bonds to form lengthy chains. These chains can be linear or circular, single-stranded or double-stranded, depending on the type of nucleic acid and its specific function.
Friedrich Miescher first used the term “nucleic acid” in 1869 after he separated a phosphorus-containing substance from cell nuclei. This discovery laid the foundation for our current understanding of these crucial molecules. Today, we recognize two main types of nucleic acids: deoxyribonucleic acid (DNA) and ribonucleic acid (RNA).
Nucleic acids are responsible for:
- Storing genetic information
- Transmitting hereditary traits from one generation to the next
- Directing protein synthesis
- Regulating gene expression
- Catalyzing biochemical reactions (in the case of some RNA molecules)
Understanding the components and structure of nucleic acids is crucial for grasping their diverse functions and their central role in life processes.
Components of Nucleic Acids
At the heart of nucleic acid structure are nucleotides, the fundamental building blocks that combine to form these complex molecules. Each nucleotide consists of three essential components:
- A pentose sugar
- A phosphate group
- A nitrogenous base
Let’s examine each of these components in detail to understand their structure and function within nucleic acids.
1. Pentose Sugar
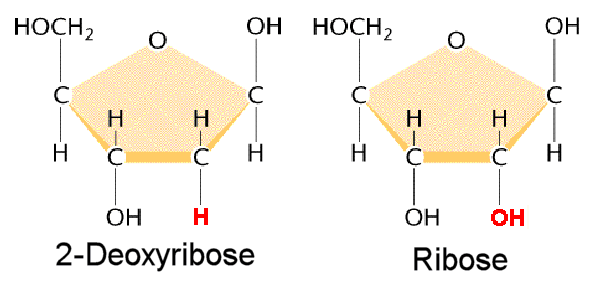
The pentose sugar in nucleotides is a five-carbon sugar molecule. The specific type of pentose sugar differs between DNA and RNA:
- In DNA, the sugar is 2-deoxyribose, which lacks an oxygen atom at the 2′ position compared to ribose.
- In RNA, the sugar is ribose.
The pentose sugar forms the backbone of the nucleic acid chain, with the phosphate groups connecting one sugar to the next. The presence of different sugars in DNA and RNA contributes to their distinct properties and functions.
2. Phosphate Group
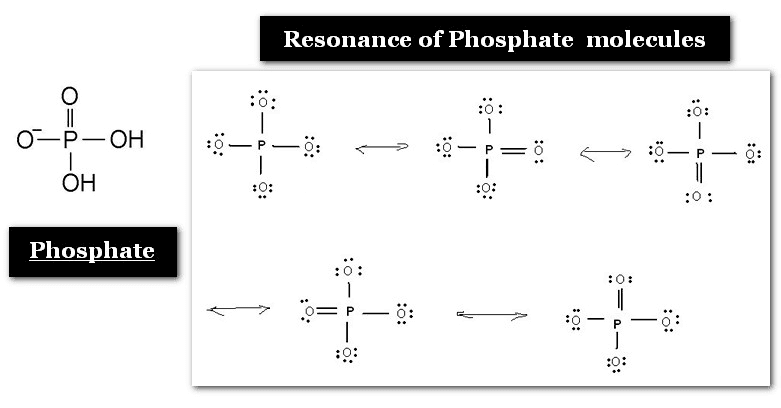
The phosphate group in nucleotides consists of a phosphorus atom bonded to four oxygen atoms. This group is crucial for forming the phosphodiester bonds that link nucleotides together in a nucleic acid chain. The phosphate groups contribute to the overall negative charge of nucleic acids, which plays a role in their interactions with other molecules and their behavior in solution.
The phosphate group is attached to the 5′ carbon of the pentose sugar in one nucleotide and the 3′ carbon of the next nucleotide to form the nucleic acid’s sugar-phosphate backbone.
3. Nitrogenous Bases
The nitrogenous bases are the components that carry the genetic information in nucleic acids. There are five main nitrogenous bases found in nucleic acids, which are divided into two categories:
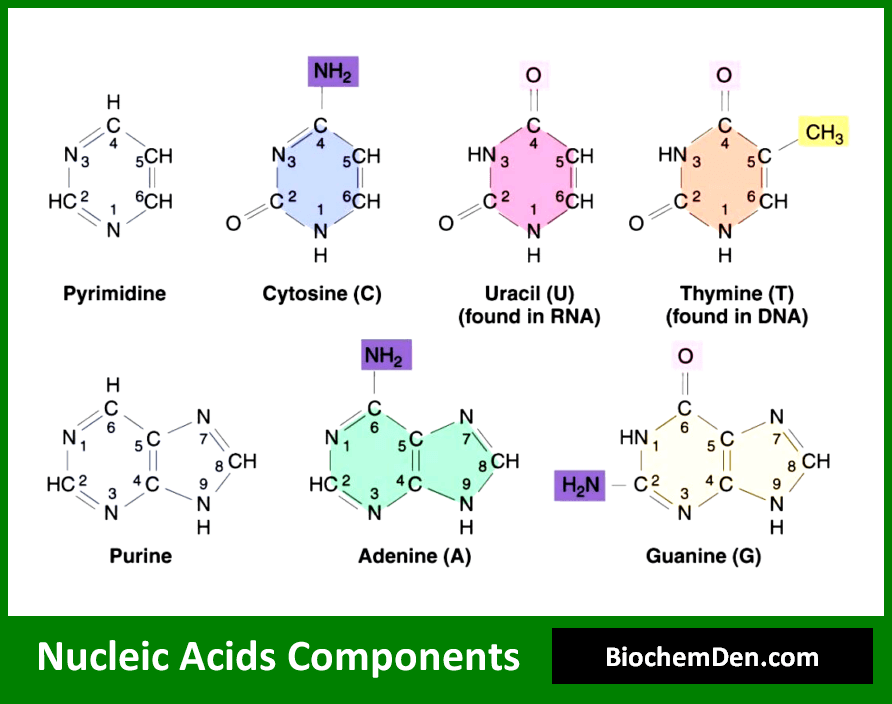
Purines:
Purine bases are found in nucleic acids and are heterocyclic compounds consisting of a pyrimidine ring and an imidazole ring fused. The two purine bases are-
- Adenine (6-Amino Purine): (C5H5N5), found in both RNA and DNA, is a white crystalline purine base, with a molecular weight of 135.15 Dalton and a melting point of 360 to 365 C.
- Guanine (2-Amino-6-oxyPurine): (C5H5ON5), also found in both DNA and RNA, is a colorless, insoluble crystalline substance, with MW=151.15 Dalton. It was first isolated from guano (bird manure), hence its name.
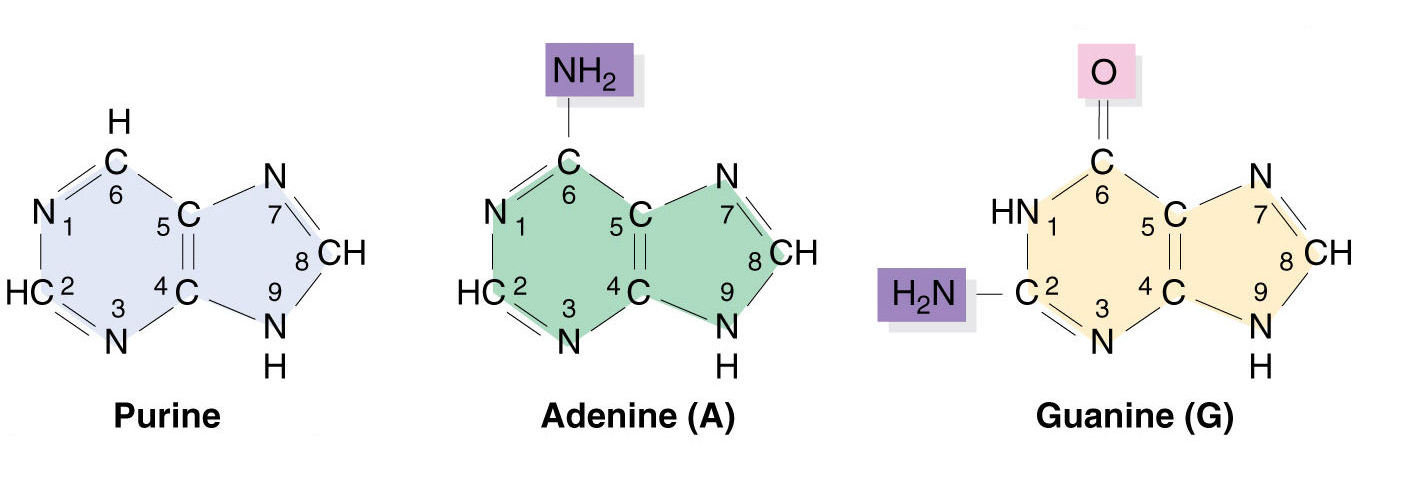
Pyrimidines:
Pyrimidine bases consist of a six-membered ring with two nitrogen atoms. The pyrimidine bases are –
- Cytosine (2-Oxy-4-aminopyrimidine) (C5H6O2N5), found in both RNA and DNA, is a white crystalline substance, with an MW of 111.12 Daltons and a melting point of 320 to 325 C.
- Thymine (2, 4-dioxy-5-methyl pyrimidine): (C5H6O2N2) found in DNA molecules only, has an MW of 126.13 Daltons. It was first isolated from the thymus, hence its name. Thymine is present in RNA only.
- Uracil (2, 4-dioxy pyrimidine): (C4H4O2N2), found in RNA molecules only, is a white, crystalline pyrimidine base with an MW of 112.10 daltons and a melting point of 338 C. Uracil is present in DNA only.
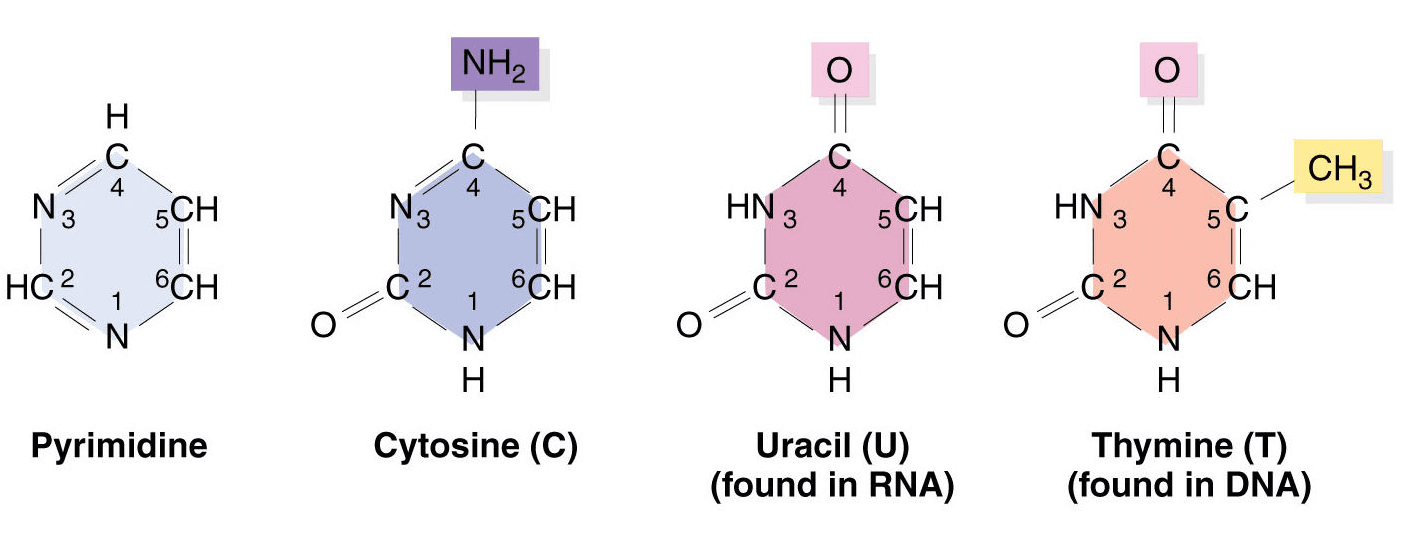
Basic Components of Nucleic Acids: Pyrimidines
These bases pair specifically with each other through hydrogen bonds:
- Adenine pairs with Thymine (in DNA) or Uracil (in RNA)
- Guanine pairs with Cytosine
The specific sequence of these bases along a nucleic acid strand encodes genetic information, determining the characteristics and functions of living organisms.
Types of Nucleic Acids
While nucleic acids share many common features, there are two main types with distinct structures and functions: DNA and RNA. Understanding the differences between these two types is crucial for grasping their roles in biological processes.
1. Deoxyribonucleic Acid (DNA)
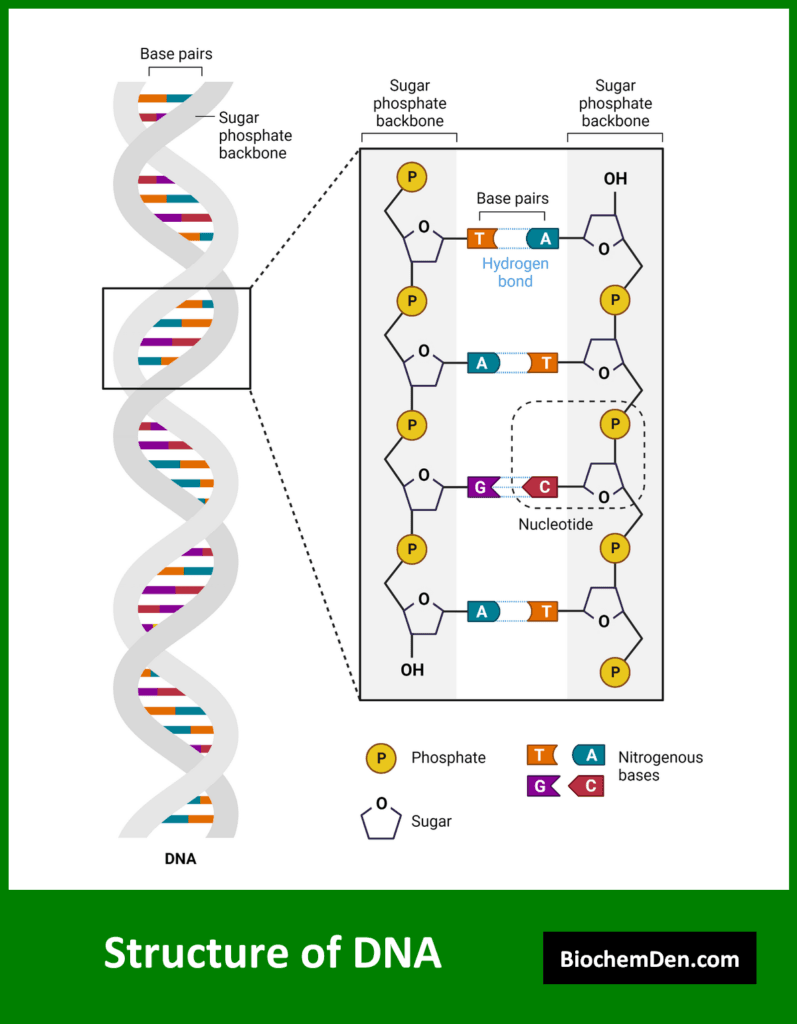
DNA is often referred to as the “blueprint of life” due to its role in storing and transmitting genetic information. Key features of DNA include:
- Sugar component: 2-deoxyribose
- Nitrogenous bases: Adenine, Guanine, Cytosine, and Thymine
- Structure: Usually double-stranded, forming the famous double helix
- Location: Primarily found in the cell nucleus, with some in mitochondria and chloroplasts
- Stability: More stable than RNA due to the absence of the 2′ hydroxyl group on the sugar
DNA’s primary function is to store genetic information over long periods of time and transmit it from one generation to the next. The sequence of bases in DNA encodes the instructions for building and maintaining an organism.
2. Ribonucleic Acid (RNA)
RNA plays a crucial role in gene expression and protein synthesis. Its key features include:
- Sugar component: Ribose
- Nitrogenous bases: Adenine, Guanine, Cytosine, and Uracil (replacing Thymine)
- Structure: Usually single-stranded, but can form complex secondary structures
- Location: Found in both the nucleus and cytoplasm
- Stability: Less stable than DNA due to the presence of the 2′ hydroxyl group on the sugar
There are several types of RNA, each with specific functions:
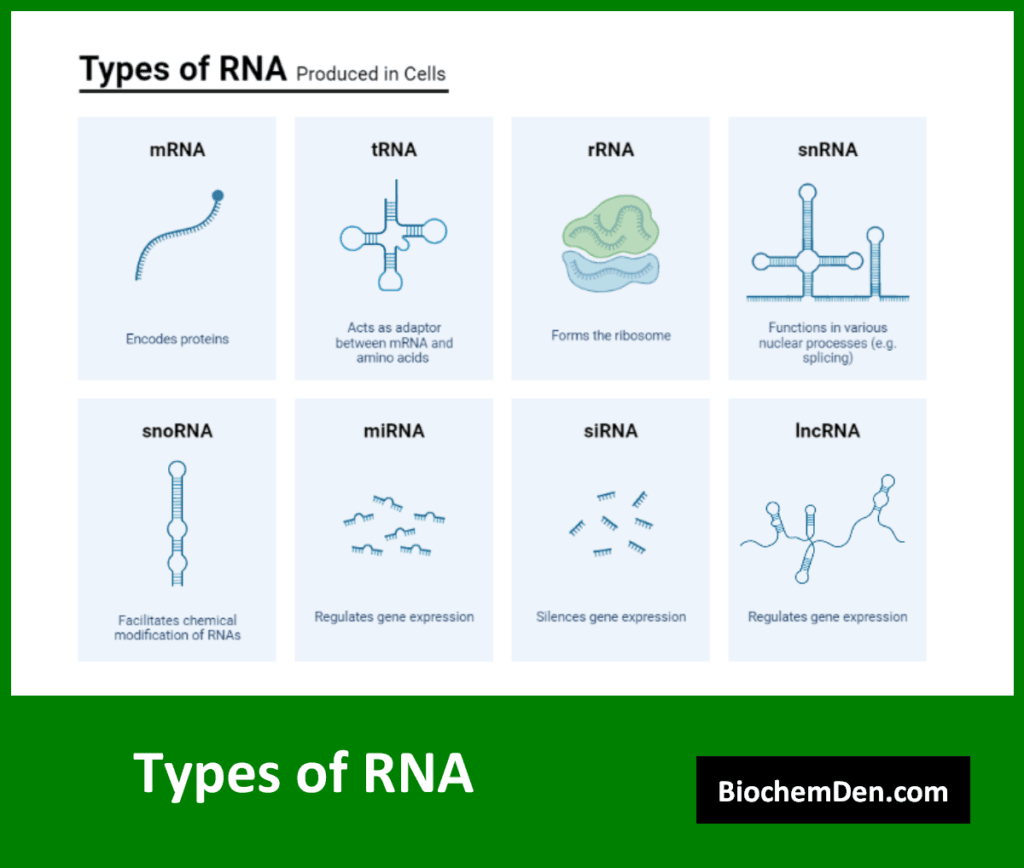
- Messenger RNA (mRNA): Carries genetic information from DNA to ribosomes for protein synthesis
- Transfer RNA (tRNA): Brings amino acids to ribosomes during protein synthesis
- Ribosomal RNA (rRNA): Forms part of the structure of ribosomes
- Non-coding RNAs: Various RNA molecules with regulatory and catalytic functions
RNA’s versatility allows it to play multiple roles in cellular processes, from carrying genetic information to catalyzing biochemical reactions.
Structure of Nucleic Acids
The structure of nucleic acids can be described at three levels: primary, secondary, and tertiary. Each level of structure contributes to the molecule’s overall function and properties.
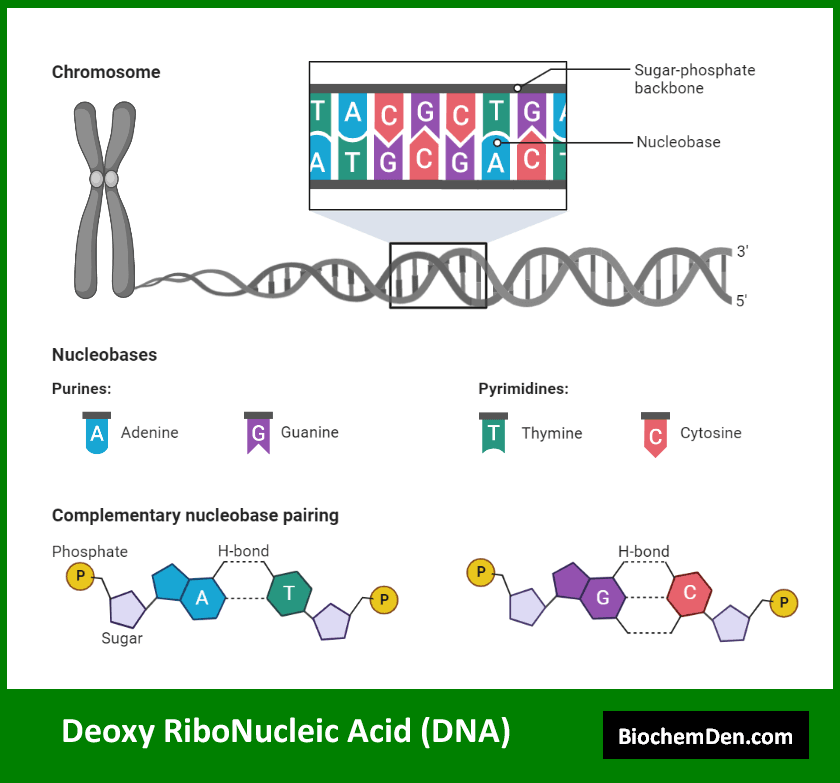
1. Primary Structure
The primary structure of a nucleic acid refers to the sequence of nucleotides along the sugar-phosphate backbone. This sequence is typically represented using the letters A, G, C, and T (or U for RNA) to denote the nitrogenous bases. The primary structure is crucial as it encodes the genetic information.
Key features of the primary structure include:
- The 5′ to 3′ directionality of the sugar-phosphate backbone
- The phosphodiester bonds linking adjacent nucleotides
- The specific sequence of nitrogenous bases
2. Secondary Structure
The secondary structure of nucleic acids describes the regular patterns formed by interactions between nucleotides. The most well-known secondary structure is the DNA double helix, first described by James Watson and Francis Crick in 1953.
DNA secondary structure:
- Two antiparallel strands wound around each other
- Base pairs held together by hydrogen bonds (A-T and G-C)
- Major and minor grooves along the helix
RNA secondary structure:
- Often forms complex patterns through intramolecular base pairing
- Common structures include hairpins, loops, and pseudoknots
3. Tertiary Structure
The tertiary structure refers to the three-dimensional arrangement of the entire nucleic acid molecule. This level of structure is particularly important for RNA molecules, which can form complex 3D shapes crucial for their functions.
Examples of tertiary structures include:
- Supercoiling of DNA in chromosomes
- Complex folding patterns in functional RNA molecules like tRNA
Understanding the various levels of nucleic acid structure is essential for comprehending how these molecules interact with other cellular components and perform their biological functions.
Functions of Nucleic Acids
Nucleic acids play central roles in numerous biological processes, from storing genetic information to regulating gene expression. The functions of nucleic acids can be broadly categorized based on whether they are performed by DNA or RNA.
1. DNA Functions
DNA serves as the primary repository of genetic information in most living organisms. Its key functions include:
- Information storage: DNA encodes the instructions for building and maintaining an organism.
- Heredity: DNA is passed from parents to offspring, transmitting genetic traits.
- Gene expression regulation: Specific DNA sequences control when and how genes are expressed.
- Replication: DNA can make copies of itself, ensuring genetic continuity across cell divisions.
- Mutation and evolution: Changes in DNA sequences drive genetic diversity and evolution.
2. RNA Functions
RNA molecules have diverse functions in cellular processes:
- Genetic information transfer: mRNA carries genetic information from DNA to ribosomes.
- Protein synthesis: tRNA and rRNA are crucial components in the translation of genetic information into proteins.
- Gene regulation: Various non-coding RNAs play roles in controlling gene expression.
- Catalysis: Some RNA molecules, like ribozymes, can catalyze biochemical reactions.
- Structural roles: rRNA forms part of the structure of ribosomes.
- Cellular defense: Some RNA molecules are involved in defending cells against viruses.
The diverse functions of nucleic acids highlight their fundamental importance in biological systems and their potential applications in biotechnology and medicine.
Nucleic Acid Synthesis
The synthesis of nucleic acids is a crucial process in all living organisms, encompassing DNA replication, transcription, and translation. These processes ensure the faithful transmission of genetic information and its expression into functional proteins.
1. DNA Replication
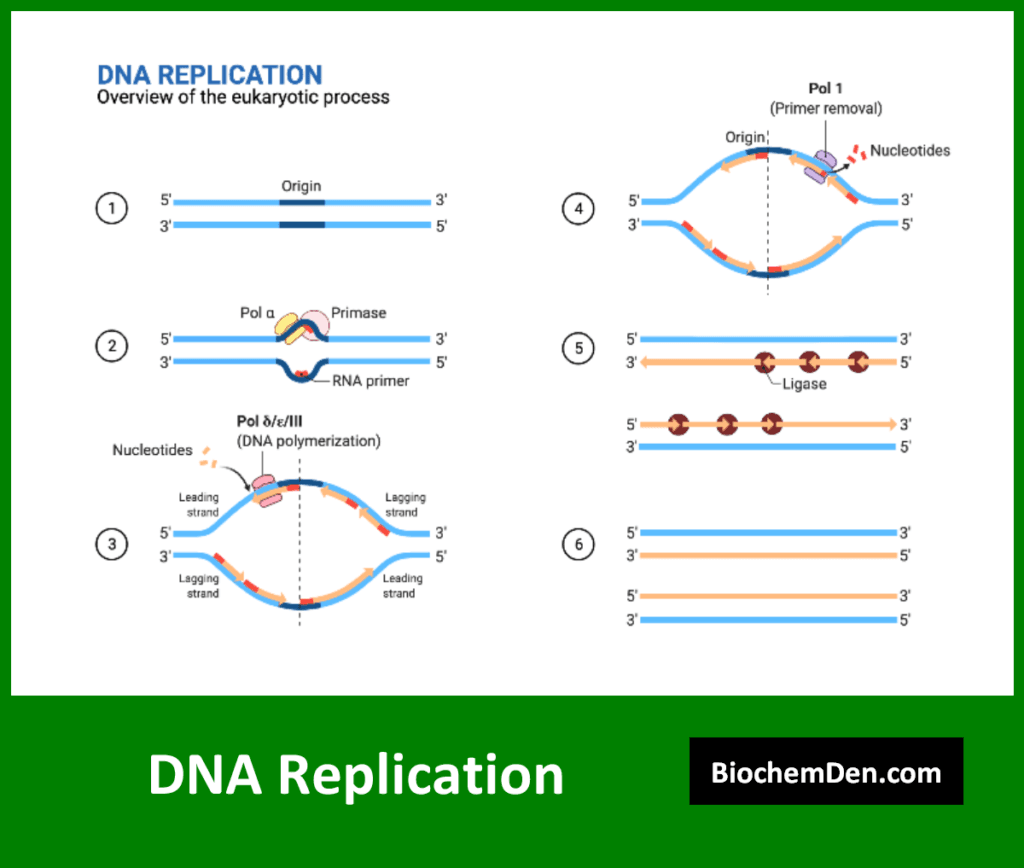
DNA replication is the process by which a cell makes an exact copy of its DNA. This process is essential for cell division and the transmission of genetic information to daughter cells. Key features of DNA replication include:
- Semi-conservative nature: Each new DNA molecule contains one original strand and one newly synthesized strand
- Involvement of multiple enzymes, including DNA polymerase, helicase, and ligase
- High fidelity, with error rates as low as 1 in 10^9 base pairs
- It occurs during the S phase of the cell cycle
The process of DNA replication involves several steps:
- Unwinding of the DNA double helix
- Formation of replication forks
- Synthesis of new DNA strands in the 5′ to 3′ direction
- Proofreading and error correction
- Ligation of Okazaki fragments on the lagging strand
2. Transcription
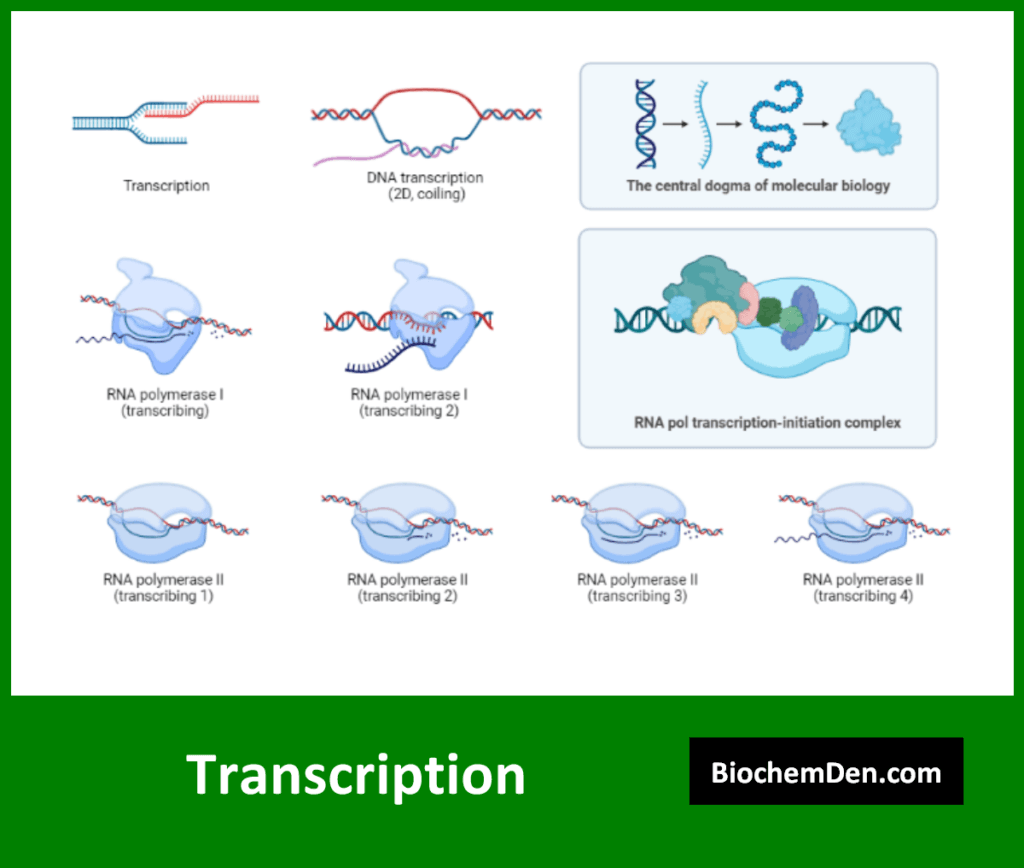
Transcription is the process by which information in DNA is copied into a complementary RNA molecule. This process is the first step in gene expression. Key aspects of transcription include:
- It occurs in the nucleus of eukaryotic cells
- Catalyzed by the enzyme RNA polymerase
- Produces various types of RNA, including mRNA, tRNA, and rRNA
- Regulated by promoter sequences and transcription factors
The transcription process can be divided into three main stages:
- Initiation: RNA polymerase binds to the promoter region
- Elongation: The RNA strand is synthesized in the 5′ to 3′ direction
- Termination: Transcription stops at specific termination sequences
In eukaryotes, the primary transcript undergoes further processing, including the addition of a 5′ cap, a 3′ poly-A tail, and splicing to remove introns.
3. Translation
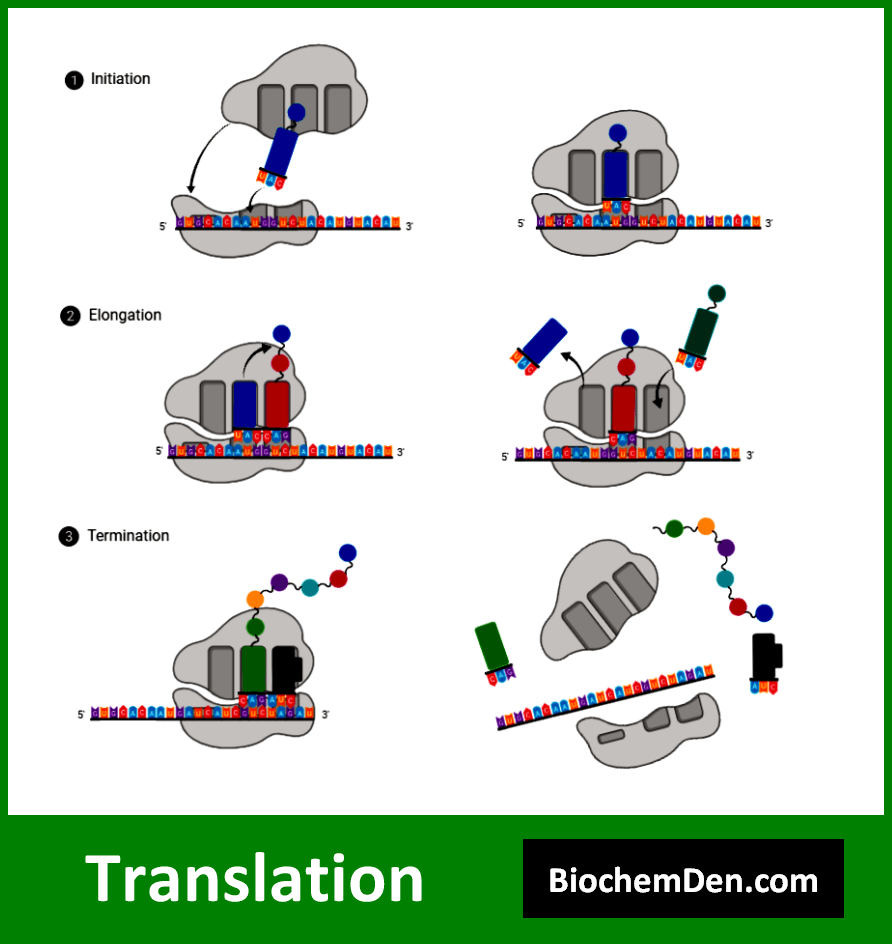
Translation is the process of converting mRNA’s genetic information into proteins. While not directly a nucleic acid synthesis process, it is the final step in converting the information stored in nucleic acids into functional proteins. Key features of translation include:
- It occurs on ribosomes in the cytoplasm
- Involves mRNA, tRNA, and various protein factors
- Uses the genetic code to convert nucleotide sequences into amino acid sequences
The translation process can be divided into three main stages:
- Initiation: The ribosome assembles on the mRNA at the start codon
- Elongation: Amino acids are added to the growing polypeptide chain
- Termination: The completed protein is released from the ribosome
Understanding these processes of nucleic acid synthesis and protein production is crucial for comprehending how genetic information is utilized in living organisms and forms the basis for many biotechnological applications.
Nucleic Acids in Modern Biotechnology
The understanding of nucleic acid structure and function has revolutionized many fields, particularly biotechnology and medicine. Some key applications of nucleic acids in modern biotechnology include:
- Genetic Engineering: Techniques like CRISPR-Cas9 allow for precise editing of DNA sequences, opening up possibilities for treating genetic disorders and improving crop yields.
- Polymerase Chain Reaction (PCR): This technique amplifies specific DNA sequences, enabling applications ranging from forensic analysis to disease diagnosis.
- DNA Sequencing: High-throughput sequencing technologies have made it possible to sequence entire genomes quickly and affordably, driving advances in personalized medicine and evolutionary biology.
- Recombinant DNA Technology: This allows for the creation of novel DNA sequences and the production of recombinant proteins, crucial for the pharmaceutical industry and research.
- RNA Interference (RNAi): This technique uses small RNA molecules to regulate gene expression, with potential applications in treating various diseases.
- Gene Therapy: The introduction of functional genes into cells to treat genetic disorders is becoming increasingly feasible thanks to advances in nucleic acid research.
- DNA Nanotechnology: Exploiting the predictable base-pairing of DNA to create nanoscale structures and devices for various applications.
- Aptamer Technology: Using short, single-stranded DNA or RNA molecules that can bind specifically to target molecules for diagnostic and therapeutic purposes.
- DNA Data Storage: Exploring the use of DNA as a high-density, long-term data storage medium.
- Synthetic Biology: Designing and constructing new biological parts, devices, and systems, or redesigning existing natural biological systems for useful purposes.
These applications demonstrate the far-reaching impact of nucleic acid research on various fields, from medicine to information technology. As our understanding of nucleic acids continues to grow, so too will the range of their practical applications.
Frequently Asked Questions (FAQs)
What are the primary components of nucleic acids?
Nucleic acids are composed of three main components: a phosphate group, a five-carbon sugar (either ribose or deoxyribose), and a nitrogenous base.
What is the role of the phosphate group in nucleic acids?
The phosphate group forms the backbone of the nucleic acid structure, linking the sugars of two adjacent nucleotides together through phosphodiester bonds.
How do the sugars in DNA and RNA differ?
DNA contains the sugar deoxyribose, which lacks one oxygen atom compared to ribose, the sugar found in RNA. This difference is crucial for the structural and functional variations between DNA and RNA.
What are the types of nitrogenous bases found in nucleic acids?
There are two types of nitrogenous bases: purines (adenine and guanine) and pyrimidines (cytosine, thymine in DNA, and uracil in RNA).
How do nitrogenous bases pair in DNA and RNA?
In DNA, adenine pairs with thymine (A-T) and guanine pairs with cytosine (G-C). In RNA, adenine pairs with uracil (A-U) and guanine pairs with cytosine (G-C).
Final words on The Building Blocks Nucleotides
Nucleic acids, with their complex structures and diverse functions, truly are the molecules of life. From the storage and transmission of genetic information to the regulation of cellular processes, these remarkable biomolecules play crucial roles in all living organisms.
In this comprehensive guide, we’ve explored the components of nucleic acids, delving into the structure of nucleotides, the differences between DNA and RNA, and the various levels of nucleic acid structure. We’ve examined the crucial functions of these molecules, from information storage to protein synthesis, and discussed the processes of nucleic acid synthesis that are fundamental to life.
Moreover, we’ve seen how our understanding of nucleic acids has revolutionized modern biotechnology, opening up new frontiers in medicine, agriculture, and even information technology. The ability to manipulate and analyze nucleic acids has given us unprecedented insight into the workings of life and powerful tools to address some of humanity’s greatest challenges.
As research in this field continues to advance, we can expect even more exciting discoveries and applications. From personalized medicine based on individual genetic profiles to the development of new therapies for previously untreatable diseases, the study of nucleic acids promises to continue shaping our world in profound ways.
Whether you’re a student beginning your journey into molecular biology, a researcher pushing the boundaries of nucleic acid science, or simply someone fascinated by the molecular basis of life, understanding the components and functions of nucleic acids provides a window into the fundamental processes that underlie all living systems.
The story of nucleic acids is, in many ways, the story of life itself. As we continue to unravel their mysteries, we gain not only a deeper understanding of biology but also powerful tools to shape the future of medicine, biotechnology, and beyond. The exploration of nucleic acids remains one of the most exciting and promising areas of scientific research, holding the potential to transform our world in ways we are only beginning to imagine.
Key Takeaways:
- Nucleic acids, primarily DNA and RNA, are the fundamental molecules of genetic information in all known life forms.
- The basic building blocks of nucleic acids are nucleotides, consisting of a pentose sugar, a phosphate group, and a nitrogenous base.
- DNA and RNA differ in their sugar component (deoxyribose vs. ribose) and one of their nitrogenous bases (thymine in DNA vs. uracil in RNA).
- The structure of nucleic acids can be described at primary, secondary, and tertiary levels, each contributing to their function.
- DNA primarily functions in information storage and transmission, while RNA has diverse roles, including protein synthesis and gene regulation.
- Nucleic acid synthesis processes like DNA replication, transcription, and translation are crucial for life.
- Understanding nucleic acids has revolutionized biotechnology, enabling techniques like genetic engineering, PCR, and gene therapy.
- Ongoing research in nucleic acids continues to open new frontiers in medicine, agriculture, and technology.
As we continue to unlock the secrets of nucleic acids, we move closer to understanding the very essence of life itself, paving the way for innovations that have the potential to reshape our world.
Discover more from Biochemistry Den
Subscribe to get the latest posts sent to your email.