Amino acids are organic compounds with amine (-NH2) and carboxyl (-COOH) functional groups, as well as an R group that is unique to each amino acid. Amino acids are the building blocks of proteins and play crucial roles in various biological processes.
A unique property of amino acids is their chirality, meaning they exist in two non-superimposable mirror-image forms called enantiomers. This article will explore the chirality of amino acids, the differences between enantiomers, and the importance of chirality in biology.
What is chirality?
Chirality comes from the Greek word for hand, “chair.” It refers to objects that are non-superimposable mirror images of each other, like your left and right hands. In chemistry, chirality applies to molecules that have the same chemical structure but differ in their 3D spatial arrangements.
For a molecule to be chiral, it must contain an asymmetric carbon atom that is bonded to four different atoms or groups of atoms. This gives rise to two possible arrangements in 3D space that are mirror images of each other.
Some key points about chirality:
- Chiral molecules are stereoisomers—compounds with the same molecular formula but different 3D structures
- The two mirror-image forms are called enantiomers
- Enantiomers have identical physical properties except for how they interact with polarized light and other chiral molecules
- Chirality is important in biology as biomolecules are chiral and exhibit specific enantiomer preference
Chirality of Amino Acids
The α-carbon atom in amino acids is linked to an amino group, a carboxyl group, a hydrogen atom, and a variable R group side chain. This gives the amino acids their central chirality.
When four different substituents are added, the α-carbon changes into an asymmetric center. This means that amino acids are made up of mirror-image pairs that cannot be put on top of each other.
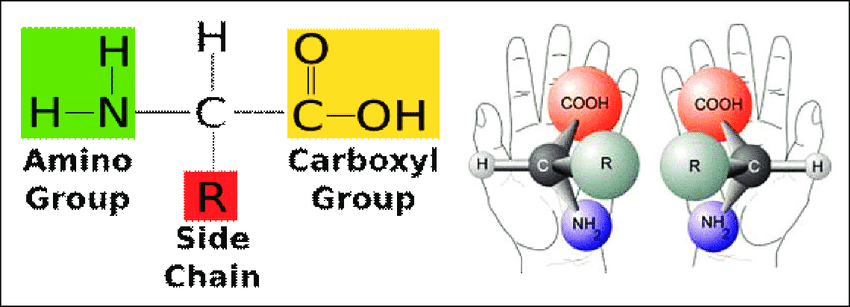
For example, the amino acid alanine contains an α-carbon bonded to -NH2, -COOH, -H, and a methyl group (-CH3). This allows for two possible arrangements in 3D space related to mirror images.
The two enantiomers of alanine are designated as:
- L-alanine: The amino group is on the left when the molecule is oriented with the carboxyl (-COOH) group pointing away.
- D-alanine: The amino group is on the right in the same orientation.
L and D designate the absolute configuration and do not relate to the optical activity. By convention, biological amino acids are of the L-configuration.
Here are some key facts about amino acid chirality:
- All amino acids except glycine are chiral with an asymmetric α-carbon.
- Natural amino acids occur in the L-configuration. D-amino acids are rare.
- Enantiomers have identical physical and chemical properties but differ in how they interact with polarized light and other chiral molecules.
- L-amino acids rotate plane polarized light to the left (levorotary). D-amino acids are dextrorotary.
- Having the correct chirality is crucial for proper protein structure and function.
Differences Between Enantiomers
Enantiomers are stereoisomers that are mirror images but non-superimposable. Though identical physically and chemically, enantiomers differ in how they interact with other chiral molecules and plane-polarized light.
Some key differences between enantiomers:
- Optical activity: Enantiomers rotate plane-polarized light in equal but opposite directions
- L-amino acids are levorotary (rotate light counter-clockwise)
- D-amino acids are dextrorotary (rotate light clockwise)
- Reactivity: Enantiomers differ in how they interact with other chiral molecules like enzymes and receptors
- L-amino acids show selective reactivity with biological systems
- D-amino acids are not readily metabolized
- Odor perception: Enantiomers can smell differently from humans. For example, L-carvone smells like spearmint, while D-carvone smells like caraway.
- Biological activity: One enantiomer displays the desired therapeutic action while the other may be inactive or even toxic. For example, L-DOPA treats Parkinson’s disease, while D-DOPA does not.
So in summary, while identical in physical properties, enantiomers differ in their biochemical interactions and biological effects due to their 3D spatial arrangements.
Importance of Chirality in Biology
Chirality is of fundamental importance in biochemistry and pharmacology. This is because:
- Biomolecules are chiral: Amino acids, sugars, nucleotides, proteins, and enzymes all exhibit chirality.
- Biological systems show stereospecificity: They preferentially recognize and utilize specific enantiomers.
- For amino acids, only L-form participates in ribosomal protein synthesis.
- Enzymes are chiral and catalyze the reactions of specific enantiomers.
- Receptors are chiral and selectively bind ligands of one enantiomer.
Some key points on chirality in biology:
- Natural amino acids are all in the L-form. D-amino acids are rare.
- L-amino acids are required for correct protein structure and function.
- Substituting D-amino acids can disrupt protein structure.
- Replacing one amino acid with its enantiomer can inactivate enzymes.
- Organisms can only metabolize and assimilate L-sugars effectively.
- Drug enantiomers exhibit differences in pharmacological activity.
So in summary, chirality produces distinct biochemical properties between enantiomers, resulting in one form being favorable for biological systems. Chirality accounts for the specificity and selectivity seen in biological interactions.
Applications of Chiral Amino Acids
The unique properties of chiral amino acids make them useful for various industrial and pharmacological applications:
- Pharmaceuticals: single-enantiomer drugs reduce adverse effects and improve efficacy. For example, L-DOPA for Parkinson’s and R-propranolol for hypertension.
- Food and agrochemical industry: L-amino acids like lysine and glutamate are used as food additives for flavor. D-amino acids act as pesticides because they are metabolically inert.
- Chiral catalysts: D-amino acids can be used to make pharmaceutical compounds asymmetrically by acting as enantioselective chiral catalysts.
- Biodegradable plastics: Polymerization of chiral amino acids produces biodegradable plastics for medical implants and drug delivery devices.
- Analytical tools: Derivatives of D-amino acids aid in the analysis and quantification of amino acids by chromatographic techniques.
In summary, the unique chirality and biochemical properties of amino acids allow their diverse applications across many fields, including medicine, agriculture, polymer chemistry, and biotechnology.
Frequently Asked Questions
Why are only L-amino acids used in proteins?
Biological systems selectively utilize L-amino acids due to their specific chirality. D-amino acids do not readily participate in ribosomal protein synthesis. The chiral environment of the ribosome facilitates the formation of peptide bonds between L-amino acids to build proteins.
What is a racemic mixture?
A racemic mixture, or racemate, is an equal mixture of two enantiomers. Due to the canceling effect of the other enantiomer’s rotation, it does not display optical activity. Biologically active compounds need to be separated into active enantiomers for optimal effect.
Can D-amino acids be incorporated into proteins?
Yes, but to a limited extent. Some D-amino acids are post-translationally incorporated into specific proteins by enzymes, such as in bacterial cell walls. However, ribosomal synthesis utilizes L-amino acids to build protein chains. Substituting D-amino acids can disrupt protein structure.
Why are chiral pharmaceuticals important?
Most drug targets, like enzymes and receptors, are chiral. Using a single chiral drug enantiomer improves safety and efficacy by optimizing desired activity and eliminating undesirable side effects from the other enantiomer.
How are enantiomers separated?
Enantiomers are physically identical, so conventional methods cannot separate them. Chiral chromatography techniques, using a chiral stationary phase, allow separation based on differences in how enantiomers interact. Other methods include chiral shift reagents, enzymatic separation, and chiral crystallization.
Key Facts and Statistics
- There are 20 common amino acids that cells use to build proteins.
- All-natural amino acids are L-isomers except glycine, which is achiral (has internal symmetry).
- D-amino acids are very rare in nature but created artificially.
- Homochirality, the exclusive use of L-amino acids, is a signature of life.
- Substituting a single L-amino acid with a D-form in a protein can disrupt its structure and function.
- 9 out of 10 new drugs in development are chiral compounds.
- The global market for chiral chemicals and pharmaceuticals was valued at USD 138.1 billion in 2021 and is predicted to grow at a CAGR of 6.8% from 2022 to 2030.
- Chiral technology (assets and expertise for chiral separation) is a key area for growth, investment, and acquisitions in pharma.
- North America accounted for the largest share (42%) of the global chiral technology market in 2021.