Peptide bonds are essential components that link together amino acids and form protein chains and polymers. Understanding peptide bonds is key to appreciating protein structure and function in fields ranging from biochemistry to pharmacology. This comprehensive guide covers everything you need to know.
“The peptide is formed between the amino group (-NH2) of the first amino acid and the carboxyl group (-COOH) of the second amino acid by eliminating one molecule of water.”
The amino acids are held together in a protein by covalent peptide bonds or linkages. These bonds are rather strong and serve as the cementing material between the individual amino acids. Peptide bonds are a type of amide bond.
What is Peptide Bond in Biology?
Peptide bond, also referred to as amide bonds, are covalent chemical bonds that form between two amino acids when the carboxyl group of one amino acid reacts with the amino group of another amino acid. This forms a special linkage where the carbon atom of one amino acid bonds with the nitrogen atom of the next amino acid, with the loss of a water molecule.
The resulting bond creates a peptide plane and chain. Chains with fewer than 50 amino acids are generally called peptides, while those with more than 50 amino acids are proteins. The sequence and number of amino acids ultimately define the protein’s three-dimensional folded structure and function.
Write about Peptide bond Structure?
Peptide bonds form the connecting links between amino acids in peptides and proteins. They form when the amine nitrogen of one amino acid reacts with the carboxyl carbon of another amino acid through a dehydration reaction, eliminating water and bonding the two acids together.
Structurally, the peptide bond links the carbon of one amino acid with the nitrogen of the adjacent amino acid. Specifically, the carbonyl carbon atom of the first amino acid bonds with the nitrogen atom on the second amino acid. The resulting bond generates a peptide group or unit.
Key features of the peptide bond structure are:
- Planarity: The atoms of the peptide bond all lie in the same plane due to resonance, giving it a planar and rigid shape.
- Rotation resistance: Single bonds between carbon atoms can usually rotate freely, but the peptide bond’s partial double bond nature limits rotation. This means it adopts a relatively fixed planar structure.
- Polarity: The bond structure reveals both polar and nonpolar character, with the electronegative oxygen pointing outward and hydrogen atoms pointing inward.
- Bond length: Average bond length is about 1.33 Angstroms. Bond angles tend to be near 110° due to surrounding atom geometry.
- Stabilization: Adding further stability, the carbonyl oxygen often participates in hydrogen bonding with nearby amide hydrogens in the chain’s backbone structure.
The peptide bond is the central structure linking amino acids together in sequence to form peptides and proteins with specific shapes and functions. Its essential structure is composed of a resonance stabilized, semi-rigid bond between the backbone carbon and nitrogen atoms of adjacent amino acids.
Peptide Bond Formula
The molecular formula of a peptide bond is:
H2N-CHR1-CO-CHR2
Where:
- H2N represents the amine group (-NH2)
- CHR1 represents the alpha carbon and side chain (R group) of the first amino acid
- CO represents the carbonyl group (C=O) of the carboxyl group
- CHR2 represents the alpha carbon and variable side chain of the second amino acid
So a peptide bond forms between the carboxyl carbon of one amino acid and the amine nitrogen of another amino acid. A covalent bond forms while eliminating water, creating the CONH amide linkage centrally found in all peptide bonds.
For example, if two glycine amino acids were linked by a peptide bond, its structure would be:
H2N-CH2-CO-NH-CH2-COOH
The atoms within the bracket [NH-CH2] represent one peptide bond unit between the glycine residues. Every amino acid linked in a peptide or protein is connected through peptide bonds. The repetitive backbone can also be illustrated as follows:
-[H2N-CHR-CO]n-
Where the carbon and nitrogen atoms forming the peptide bond are highlighted in brackets. The R refers to diverse amino acid side chains protruding off the backbone chain at each alpha carbon. And n refers to the number of peptide bonds occurring in series along the molecule. This represents the shared basic structure found in all polypeptides and proteins.
Key Properties of Peptide Bonds
Peptide bonds share the following key properties:
- They are rigid and planar, holding the backbone atoms in a single plane. This creates local rigidity.
- They cannot rotate freely. This is due to resonance contributing to partial double bond character.
- They are polar but relatively hydrophobic. The electronegative oxygen points away from the bond’s axis.
- They are moderately stable, with an energy content of about 5–10 kcal/mol. However, the bonds preceding peptide bonds can rotate much more freely.
Peptide Bond Formation – Step by Step
Peptide bond formation follows these key steps:
- An amino group from one amino acid lines up near the carboxyl group of another amino acid. This reaction occurs due to the loss of water and condensation reaction.
- The carbon within the carboxyl group bonds with the nitrogen in the amino group. This creates the peptide bond between the carbon and nitrogen atoms.
- The electrons in the bond are delocalized across both nitrogen and oxygen atoms, which causes the peptide bond to resist further rotation. This creates a planar peptide group.
- Multiple amino acids can now keep bonding to elongate the peptide chain. The sequence of amino acids in the chain define the protein’s structure and properties.
When the amino group of an amino acid combines with the carboxyl group of another amino acid, a peptide bond is formed.
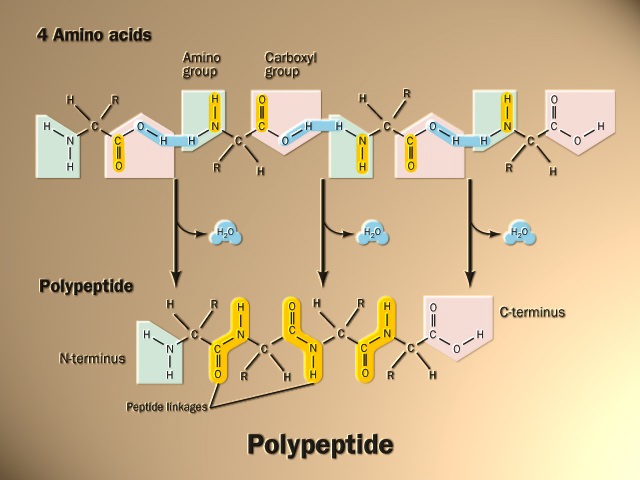
Peptide Bond Example
Alanine and glycine are two common amino acids found in peptides and proteins. The molecular structures before peptide bond formation would be:
Alanine: H2N-CH(CH3)-COOH
Glycine: H2N-CH2-COOH
To form a peptide bond between alanine and glycine:
- The carboxyl group (-COOH) of alanine reacts with the amino group (-NH2) of glycine.
- A water molecule (H2O) is released as the peptide bond forms, connecting the carbon of alanine to the nitrogen of glycine.
- This results in a dipeptide consisting of alanine and glycine joined by a peptide bond:
H2N-CH(CH2)-CONH–CH2–COOH
In this structure, the atoms highlighted:
-[CONH–CH2]-
represent a single peptide bond between alanine and glycine. This same process occurs repeatedly as amino acids get added into growing peptide chains through peptide bond formation.
So in summary, a peptide bond has formed between the two amino acids, leaving an amine at the beginning and a carboxyl group at the end for further elongation with additional amino acids. This exemplifies the typical peptide bond linkage found between every amino acid in all peptides and proteins.
Depending on the number of amino acids molecules composing a chain, the peptide may be termed as
- Dipeptide = containing 2 amino acid units
- Tripeptide = containing 3 amino acid units
- Tetrapeptide = containing 4 amino acid units
- Oligopeptide = containing not more than 10 amino acid units
- Polypeptide = containing more than 10 amino acid units, up to 100 residues
- Macropeptides = made up of more than 100 amino acids
Salient features of Peptide bond
- The peptide bond is rigid and planar
- The atoms in the peptide bond are Cα-C-N-Cα.
- The peptide bond is coplanar, this indicated a resonance or partial sharing of two pairs of electrons between the carbonyl oxygen and the amide nitrogen.
- The 4 atoms of the peptide group (C, H, O, and N) lie in a single plane, in such a way that the oxygen atom of the carbonyl group and the hydrogen atom of the amide nitrogen are trans to each other.
- The peptide bond shows a partial double bond character.
Peptide Bonds Role in Protein Structure
The rigidity and planar orientation of peptide bonds generates stretches within protein chains that form spiral shapes or pleated sheets. Non-polar, hydrophobic amino acids collapse inward away from water, while charged hydrophilic amino acids on the protein surface interact with surrounding water molecules and ions.
Ultimately, the sequence order of amino acids dictates the complex folds and higher orders of protein structure. Peptide bonds establish primary structure while non-covalent interactions among amino acid side chains guide higher order folding which defines function. Breaking peptide bonds denatures and inactivates proteins.
Characteristics of Peptide bond
The peptide bond is rigid and planar, with a partial double bond in character. It generally exists in trans-configuration. Both –C=O and –NH groups of peptide bonds are planar and are involved in hydrogen bond formation.
1. Writing of Peptide structures (or) N and C-terminals
Conventionally, the peptide chains are written with the free amino end (N-terminal residue) at the left, and the free carboxyl end (C-terminal residue) at the right. The amino acid sequence is read from the N-terminal end to the C-terminal end. Incidentally, protein biosynthesis also starts from the N-terminal amino acid.
2. Representation of Peptide chain
To represent the peptide structure, we must write in “Rattle Snake moving representation” from left to right across the page. The C-terminal residues from its fangs and the N-terminal residues from its rattle.
3. Shorthand to read peptides
The amino acids in a peptide or protein are represented by the 3-letter or one-letter abbreviation. This is the chemical shorthand to write proteins.
4. The naming of Peptides
For naming peptides, the amino acid suffixes –ine (glycine), -an (tryptophan), -ate (glutamate) are changed to –yl with the exception of C-terminal amino acid. Thus, a tripeptide composed of N-terminal glutamate, a cysteine, and a C-terminal glycine is called glutamyl-cysteinyl-glycine.
5. Stereochemistry of peptide chains
All proteins are made of amino acids of L-configuration. This fixes the steric arrangement at the α-carbon atom. The dimensions of the peptide chain are known exactly.
The Peptide Bond Is Rigid and Planar
Covalent bonds also place important constraints on the conformation of a polypeptide. In the late 1930s, Linus Pauling and Robert Corey embarked on a series of studies that laid the foundation for our present understanding of protein structure. They began with a careful analysis of the peptide bond.
The α-carbons of adjacent amino acid residues are separated by three covalent bonds, arranged as Cα-C-N-Cα.
The peptide C-N bond is somewhat shorter than the C-N bond in a simple amine, and the atoms associated with the peptide bond are coplanar, according to X-ray diffraction investigations of crystals of amino acids, simple dipeptides, and tripeptides.
This indicated a resonance or partial sharing of two pairs of electrons between the carbonyl oxygen and the amide nitrogen.
The oxygen has a partial negative charge and the nitrogen has a partial positive charge, setting up a small electric dipole.
The six atoms of the peptide group lie in a single plane, with the oxygen atom of the carbonyl group and the hydrogen atom of the amide nitrogen Trans to each other.
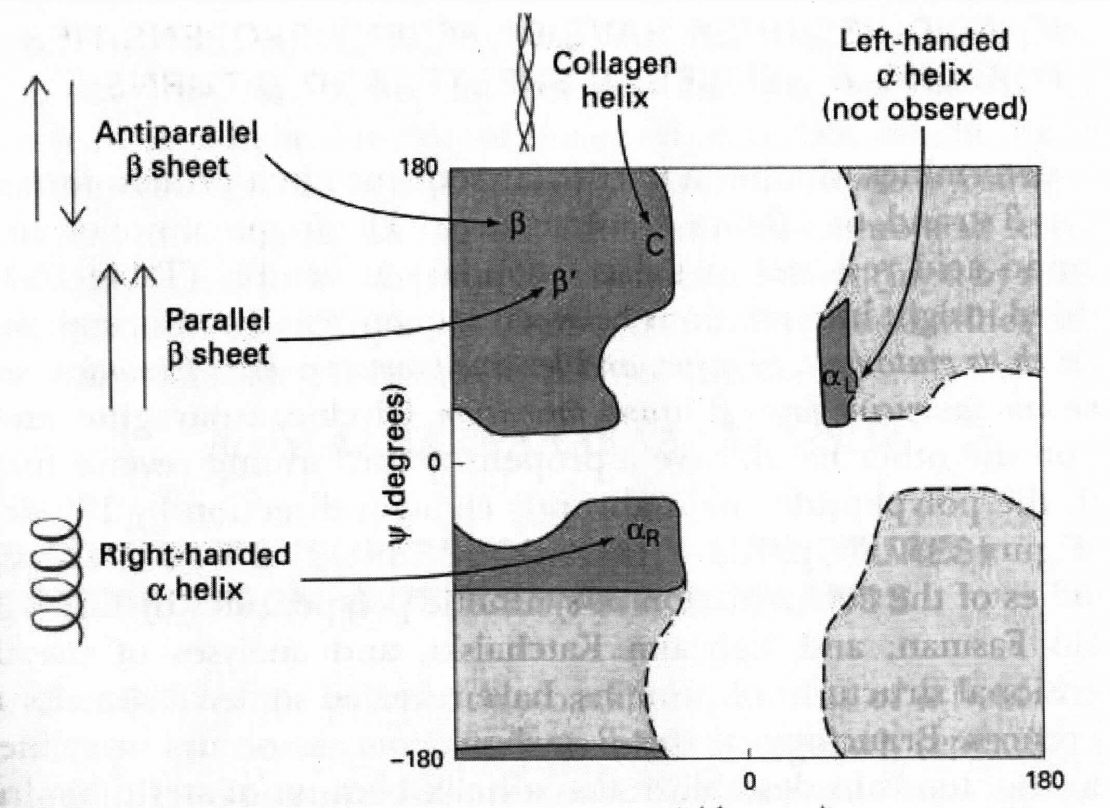
From these findings, Pauling and Corey concluded that the peptide C-N bonds are unable to rotate freely because of their Partial double-bond character. Rotation is permitted about the N-Cα and the Cα -C bonds. The backbone of a polypeptide chain can thus be pictured as a series of rigid planes, with consecutive planes sharing a common point of rotation at Cα. The rigid peptide bonds limit the range of conformations that can be assumed by a polypeptide chain.
By convention, the bond angles resulting from rotations at Cα are labeled Φ (phi) for the N-Cα bond and ψ (psi) for the Cα-C bond. Again, by convention, both Φ and ψ are defined as 1800 when the polypeptide is in its fully extended conformation and all peptide groups are in the same plane.
In principle, Φ and ψ can have any value between +180 and -1800, but many values are prohibited by steric interference between atoms in the polypeptide backbone and amino acid side chains.
The conformation in which both Φ and ψ are 00 is prohibited for this reason.
This conformation is used merely as a reference point for describing the angles of rotation.
Allowed values for Φ and ψ are graphically revealed when ψ are plotted versus Φ in a Ramachandran plot, introduced by G. N. Ramachandran (Gopalasamudram Narayan Ramachandran).
Key Applications of Peptide Bonds
Because peptide bonds form the basis of all proteins, their applications across biochemistry are immense including:
- Structural proteins like collagen that offer mechanical support and strength
- Enzymes that catalyze and accelerate chemical reactions essential for metabolism
- Messenger proteins like hormones that coordinate function through cell signaling
- Transporters and membrane channels that actively move ions and molecules across cell membranes
- Antibodies central to immune defense against pathogens
- Contractile muscle proteins including actin and myosin that generate movement
Therapeutic applications are also wide-ranging. Many drugs derive from proteins and peptides, as well as their synthetic analogues, that target diseases through diverse mechanisms. Examples include insulin for diabetes and monoclonal antibodies for fighting cancer or autoimmune disorders. Researchers develop novel peptides as research tools to probe protein function and as therapeutic leads tackling previously untreatable conditions.
Frequently Asked Questions (FAQs)
What holds peptide bonds together?
Peptide bonds form covalent bonds, meaning they share electron pairs between the nitrogen and carbon atoms that make up the bond itself. This forms a stable molecular orbital. Additional hydrogen bonds between carbonyl oxygens of peptide bonds and hydrogens on nearby amide nitrogens further stabilize structure.
What is unique about peptide bonds?
Peptide bonds cannot rotate like single covalent bonds due to resonance of electrons across the oxygen, carbon, nitrogen and hydrogen atoms involved. This resonance leads to partial double bond character and a planar confirmation resisting free rotation typically seen in single bonded carbon atoms.
Can peptide bonds break down?
While stable under normal conditions, peptide bonds constantly break down and reform during protein turnover. Enzymes called proteases specifically catalyze peptide bond breakdown, clipping long protein chains into smaller fragments. Changes in temperature, pH, radiation levels or chemical modifiers can break bonds through denaturation as well.
What is a peptide unit?
A peptide unit, also called a monomer unit, consists of a single amino acid that has formed peptide bonds with two other amino acids, leaving free amino and carboxyl groups on either end. Chains of many peptide units form peptide chains and eventual complete proteins.
Are lipids capable of forming peptide bonds?
No. Lipids consist of fatty acids and serve entirely different functions from peptides and proteins. They lack amino groups and cannot form peptide bonds. Proteins and peptides both contain chains of amino acids linked by peptide bonds, whereas lipids self-assemble into separate structures independent of peptide bonds.
Final words on Peptide Bonds
Through this deep dive into their structure, function and applications, it’s clear peptide bonds represent a fundamental nexus across biochemistry, cell biology and pharmacology.
From driving protein folding to enabling enzyme function to forming the basis of novel therapeutics, understanding peptide bonds provides insight across the life sciences and medicine.
Discover more from Biochemistry Den
Subscribe to get the latest posts sent to your email.