Glycolysis means “splitting sugars.”. In Glycolysis, Glucose (a six-carbon sugar) is split into two molecules of a three-carbon sugar. This pathway is also called the “Embden-Myerhof-Parnas pathway (EMP pathway)”. Glycolysis yields two molecules of ATP (free energy-containing molecule), two molecules of pyruvic acid, and two “high energy” electron-carrying molecules of NADH.
The glycolysis pathway can occur with or without oxygen. Glycolysis is the first stage of cellular respiration in the presence of oxygen. Without oxygen, Glycolysis allows cells to make small amounts of ATP. This process is called fermentation.
Cellular respiration is the process through which cells generate energy through ATP. This ATP fuels all the vital biochemical reactions that allow the cell to function and survive. The first step in cellular respiration is a metabolic process known as Glycolysis. Glycolysis is an ancient and highly conserved process present in nearly all organisms. In this pathway, Glucose is broken down to produce pyruvate through the generation of ATP and NADH. The pyruvate can continue through the complete citric acid cycle for further ATP production. Alternatively, the pyruvate can be converted to lactate or ethanol under anaerobic conditions.
This comprehensive guide will demystify Glucose metabolism —its definition, purpose, key players, individual steps, energy generation, regulation, and importance. Understanding the intricacies of this fundamental metabolic pathway provides key insights into energy production in both health and disease.
What is Glycolysis?
Glycolysis is the sequence of enzymatic reactions that metabolizes one molecule of Glucose into two pyruvate molecules with the simultaneous production of ATP and NADH. The Glucose glycolytic pathway is derived from the Greek words “glyco,” meaning sweet, and “lysis,” meaning splitting. It is an appropriate name since this pathway involves sugar (Glucose) splitting.
Glycolysis takes place in the cytoplasm of both prokaryotic and eukaryotic cells. It does not require oxygen to proceed and, therefore, can occur under aerobic and anaerobic respiration conditions. It makes Glucose catabolism the most essential and universal energy-producing pathway in various organisms.
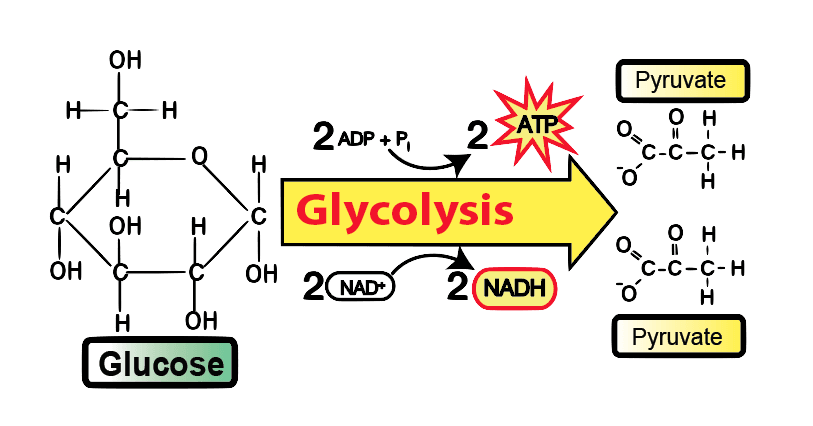
Glycolysis occurs in the cytosol of cells. Under aerobic conditions, pyruvate derived from Glucose will enter the mitochondria to undergo oxidative phosphorylation. Anaerobic respiration conditions result in pyruvate staying in the cytoplasm and being converted to lactate by the enzyme lactate dehydrogenase.
Anaerobic Glycolysis produces 2 ATP molecules per glucose molecule. Under aerobic conditions, the pyruvate generated can continue the Krebs cycle, optimizing ATP production to 32–34 molecules per glucose molecule.
Glucose as fuel
The primary function of carbohydrates in metabolism is as a fuel to be oxidized and provide energy for other metabolic processes. Carbohydrate is utilized by cells mainly as Glucose. The three principal monosaccharides resulting from digestive processes are Glucose, fructose, and galactose. Much of the Glucose is derived from starch, which accounts for almost half of the fuel in the diets of most humans.
Glucose is also produced from other dietary components by the liver and, to a lesser extent, by the kidneys. Fructose results from a large intake of sucrose, while galactose is produced when lactose is the principal carbohydrate of the diet.
Both fructose and galactose are easily converted to Glucose by the liver. Thus, glucose is the primary fuel of most organisms and can be quickly metabolized from glycogen stores when there is a sudden need for energy.
This conversion is necessary for the production of ATP, without which the cells cannot survive. Glycolysis occurs in the cytoplasm of all cells. It can occur in either the presence or absence of oxygen.
Glucose transporters
Glucose transporters are glucose-transporting proteins. Usually, Glucose is transported into the cells by a sodium-glucose pump. In addition to the transport pump, most cells have another type of transport protein called Glucose transporters (GLUT).
So far, six types of GLUT have been identified (GLUT 1–5 and 7). Among these, GLUT-4 is insulin-sensitive and is located in cytoplasmic vesicles. It is present in large numbers in muscle fibers and adipose cells.
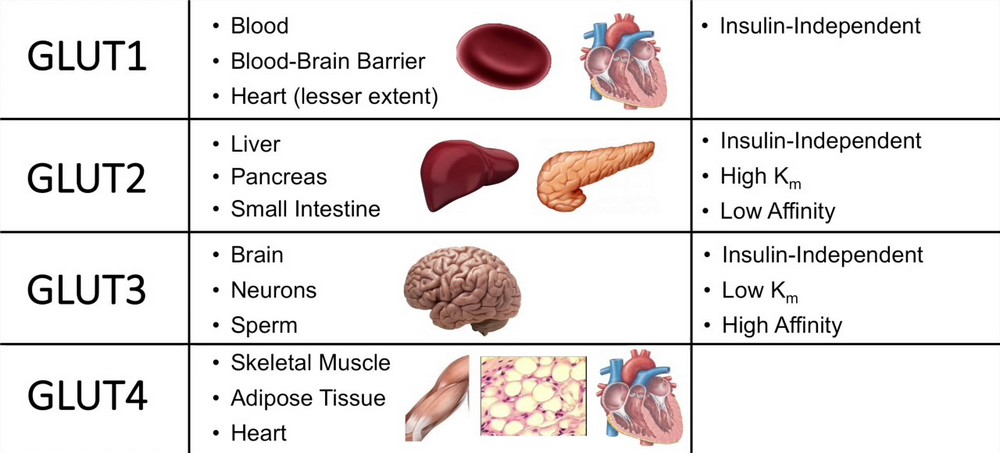
When the insulin-receptor complex is formed in the membrane of such cells, the vesicles containing GLUT-4 are attracted towards the membrane, and GLUT-4 is released into the membrane. Now, GLUT-4 starts transporting the glucose molecules from ECF into the cell. The advantage of GLUT-4 is that it transports Glucose at a faster rate.
Key Players in Glycolysis
Glycolysis, a crucial metabolic pathway, occurs in the cytoplasm of cells and is fundamental to meeting cellular energy requirements. It consists of a series of enzymatic reactions that convert Glucose into intermediates.
Enzymes of Glycolysis play a pivotal role in regulating each step of this pathway, ensuring the efficient conversion of Glucose into energy-rich molecules. As Glucose degradation progresses, it extracts energy for cellular metabolism, providing the necessary ATP molecules to fuel various cellular processes.
Glycolysis has two phases: the preparatory phase, which consumes ATP, and the payoff phase, which generates ATP—specific enzymes involved in Glycolysis in each step.
Preparatory Phase Enzymes
- Hexokinase: Phosphorylates glucose to glucose-6-phosphate using ATP. This irreversible step traps glucose inside the cell.
- Phosphoglucose isomerase: converts glucose-6-phosphate to fructose-6-phosphate.
- Phosphofructokinase: Phosphorylates fructose-6-phosphate to fructose-1,6-bisphosphate using another ATP molecule. It is the committed and highly regulated rate-limiting step of Glycolysis.
Payoff Phase Enzymes
- Aldolase: This enzyme breaks down fructose-1,6-bisphosphate into two 3-carbon molecules: glyceraldehyde-3-phosphate and dihydroxyacetone phosphate.
- Triosephosphate isomerase: converts dihydroxyacetone phosphate into glyceraldehyde-3-phosphate.
- Glyceraldehyde-3-phosphate dehydrogenase: oxidizes glyceraldehyde-3-phosphate into 1,3-bisphosphoglycerate while reducing NAD+ to NADH.
- Phosphoglycerate kinase: transfers a phosphate group from 1,3-bisphosphoglycerate to ADP to generate ATP.
- Phosphoglycerate mutase: converts 3-phosphoglycerate to 2-phosphoglycerate.
- Enolase: Converts 2-phosphoglycerate to phosphoenolpyruvate (PEP).
- Pyruvate kinase: transfers the phosphate group from PEP to ADP, generating pyruvate and ATP.
In addition to these enzymes, Glycolysis requires ATP and the electron carrier NAD+ as co-factors.
The Key Steps and Phases of Glycolytic Pathway
Glycolysis is a metabolic pathway and an anaerobic energy source that has evolved in nearly all types of organisms. Glycolysis can be broadly divided into two phases consisting of 5 steps each.
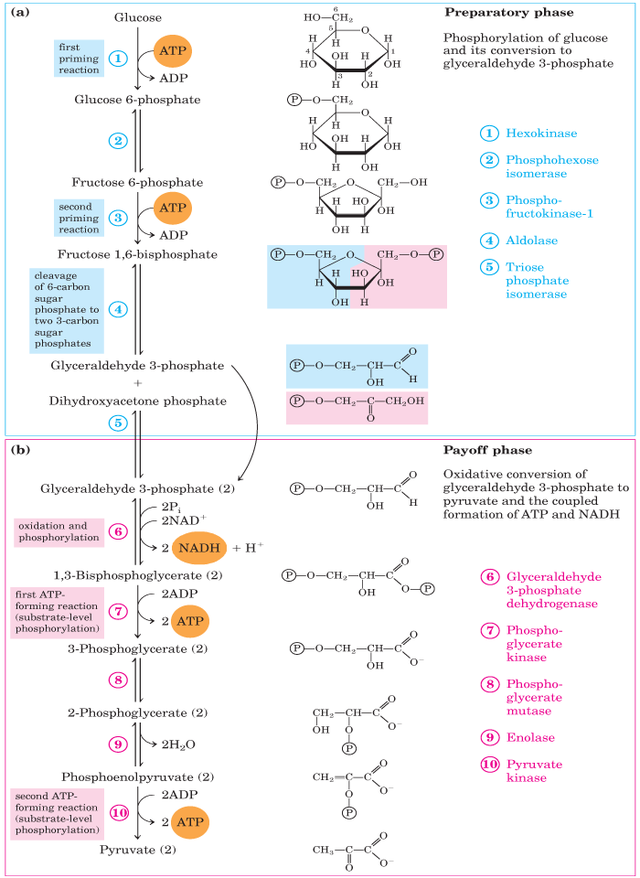
The pathway is divided into two phases
- Preparative phase (Steps 1 to 5)
- Payoff Phase (Steps 6 to 10)
Here is a detailed breakdown:
Preparatory Phase
It is the first phase of Glycolysis. Glucose is converted into glyceraldehyde-3-phosphate and DHAP (dihydroxyacetone phosphate). This phase contains five steps.
1. Glucose Phosphorylation
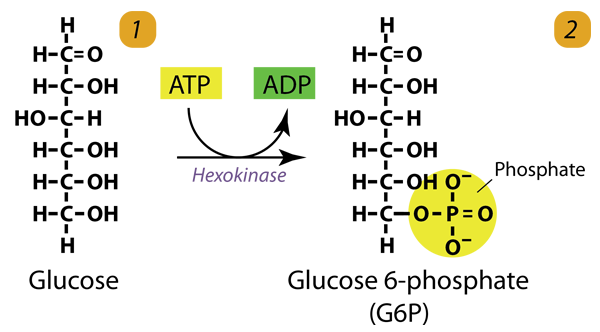
The hexokinase phosphorylates (adds a phosphate group to) Glucose in the cell’s cytoplasm. In the process, a phosphate group from ATP is transferred to Glucose, producing glucose-6-phosphate. Hexokinase transfers a phosphate group from ATP to Glucose to generate glucose-6-phosphate. This step prevents Glucose from leaving the cell.
Glucose (C6H12O6) + hexokinase + ATP → ADP + Glucose 6-phosphate (C6H11O6P1)
2. Isomerization
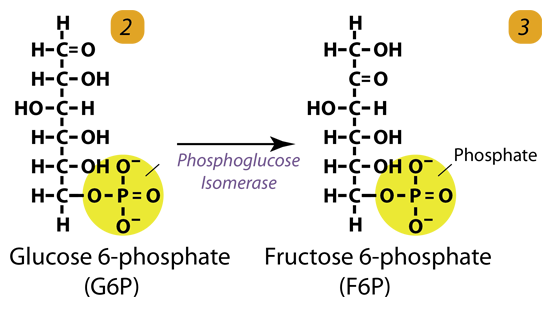
Glucose-6-phosphate is converted to fructose-6-phosphate by phosphoglucose isomerase. Isomers have the same molecular formula, but the atoms of each molecule are arranged differently.
Glucose 6-phosphate (C6H11O6P1) + Phosphoglucoisomerase → Fructose 6-phosphate (C6H11O6P1)
3. Second Phosphorylation
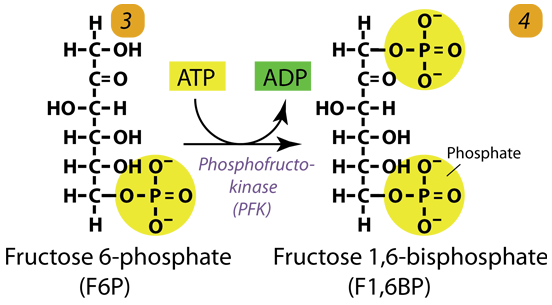
Phosphofructokinase uses another ATP to phosphorylate fructose-6-phosphate into fructose-1,6-bisphosphate, the key regulated and committed step of Glucose metabolism.
Fructose 6-phosphate (C6H11O6P1) + Phosphofructokinase + ATP → ADP + Fructose 1, 6-bisphosphate (C6H10O6P2)
4. Cleavage/Condensation
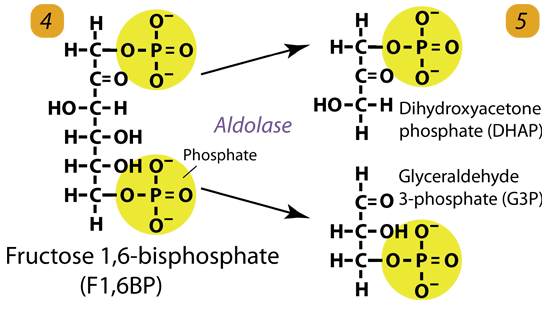
Fructose-1,6-bisphosphate is cleaved by aldolase to form two 3-carbon molecules, glyceraldehyde-3-phosphate and dihydroxyacetone phosphate.
Fructose 1, 6-bisphosphate (C6H10O6P2) + aldolase → Dihydroxyacetone phosphate (C3H5O3P1) + Glyceraldehyde phosphate (C3H5O3P1)
5. Isomerization
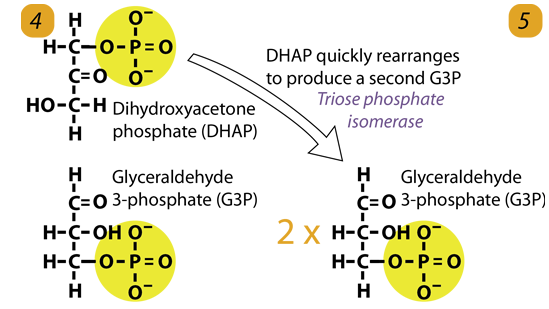
The enzyme triose phosphate isomerase rapidly interconverts the molecules dihydroxyacetone phosphate (DHAP) into glyceraldehyde-3-phosphate. Glyceraldehyde phosphate is removed as soon as it is formed for use in the next step of Glycolysis.
Dihydroxyacetone phosphate (C3H5O3P1) → Glyceraldehyde phosphate (C3H5O3P1)
The net result for steps 4 and 5:
Fructose 1, 6-bisphosphate (C6H10O6P2) ↔ 2 molecules of Glyceraldehyde phosphate (C3H5O3P1)
Payoff Phase
It is the second phase of Glycolysis. In this phase, Glyceraldehyde-3-Phosphate is converted into Pyruvate molecules. , this is the second half of Glycolysis. This phase has five steps.
6. ATP Generation / Dehydrogenation
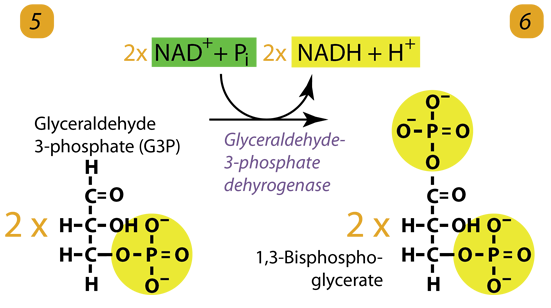
The enzyme triose phosphate dehydrogenase serves two functions in this step. First, the enzyme transfers hydrogen (H–) from Glyceraldehyde-3-phosphate to the oxidizing agent Nicotinamide Adenine Dinucleotide (NAD+) to form NADH. Next, Triose phosphate dehydrogenase adds a phosphate (P) from the cytosol to the oxidized glyceraldehyde-3-phosphate to form 1, 3-bisphosphoglycerate. It occurs for both molecules of glyceraldehyde-3-phosphate produced in step 5.
A. Triose phosphate dehydrogenase + 2 H– + 2 NAD+ → 2 NADH + 2 H+
B. Triose phosphate dehydrogenase + 2 P + 2 glyceraldehyde phosphate (C3H5O3P1) → 2 molecules of 1,3-bisphosphoglycerate (C3H4O4P2)
7. Second ATP Generation / Substrate level Phosphorylation
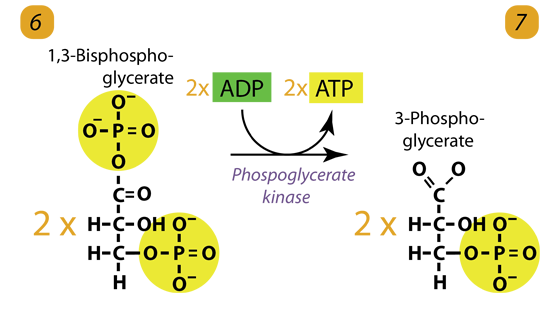
The enzyme phosphoglycerokinase transfers a P from 1,3-bisphosphoglycerate to a molecule of ADP to form ATP. It happens for each molecule of 1,3-bisphosphoglycerate. The process yields two 3-phosphoglycerate molecules and two ATP molecules.
1,3-bisphosphoglycerate generates 2,3-bisphosphoglycerate, an allosteric hemoglobin regulator, in red blood cells.
2 molecules of 1,3-bisphoshoglycerate (C3H4O4P2) + phosphoglycerokinase + 2 ADP → 2 molecules of 3-phosphoglycerate (C3H5O4P1) + 2 ATP
8. Isomerization / Dehydration
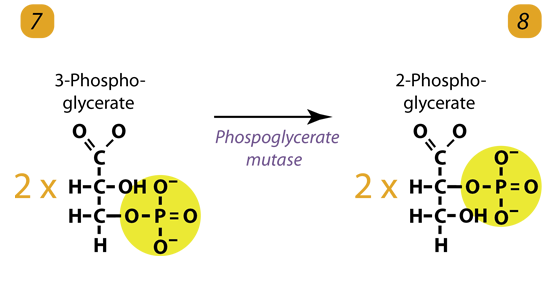
The enzyme phosphoglyceromutase relocates the PhosphatePhosphate from 3-phosphoglycerate from the third carbon to the second carbon to form 2-phosphoglycerate. Simply Phosphoglycerate mutase shifts the PhosphatePhosphate to form 2-phosphoglycerate.
2 molecules of 3-Phosphoglycerate (C3H5O4P1) + phosphoglyceromutase → 2 molecules of 2-Phosphoglycerate (C3H5O4P1)
9. Dehydrogenation / Enolyzation
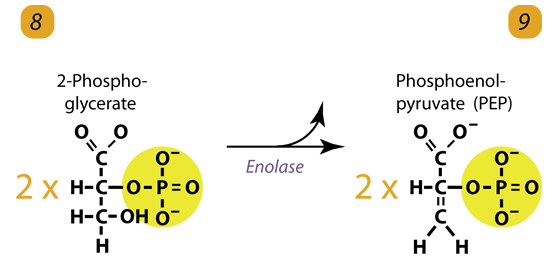
Enolase removes a water molecule from 2-phosphoglycerate to form Phospho Enol Pyruvic acid (PEP). It happens for each molecule of 2-phosphoglycerate.
2 molecules of 2-Phosphoglycerate (C3H5O4P1) + enolase → 2 molecules of phosphoenolpyruvic acid (PEP) (C3H3O3P1)
10. Substrate-Level Phosphorylation
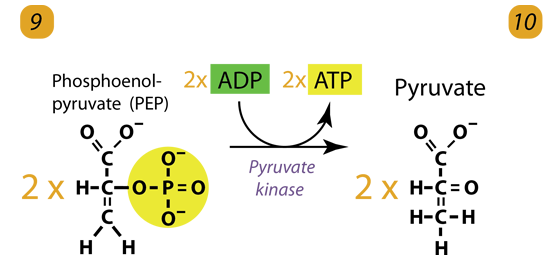
The enzyme Pyruvate kinase (PK) transfers a Phosphate from PEP to ADP to form Pyruvic acid and ATP. It happens for each molecule of PEP. This reaction yields two Pyruvate molecules and 2 ATP molecules. Pyruvate Kinase is a Potassium potassium-containing enzyme. Pyruvate kinase transfers the PhosphatePhosphate from PEP to ADP, forming pyruvate and ATP. It is irreversible and is, therefore, another key regulatory step.
2 molecules of PEP (C3H3O3P1) + pyruvate kinase + 2 ADP → 2 molecules of pyruvic acid (C3H4O3) + 2 ATP
The overall result in terms of molecules produced during the chemical breakdown of Glucose by Glycolysis.
One crucial part of Glycolysis involves releasing energy-rich molecules due to various chemical reactions in Glycolysis. These energy-rich molecules are released in this process and play a significant role in powering cellular processes, especially under anaerobic conditions.
Energy Production in Glycolysis
Glycolysis is a series of key steps that converts Glucose into intermediate molecules within the cell’s cytoplasm. A set of specific enzymes catalyzes this pathway of Glycolysis, each playing a crucial role in regulating the reactions in Glucose catabolism. Glycolysis begins when Glucose, a vital energy source, enters the glycolytic pathway.
This initial step is tightly controlled and regulated to meet the cell’s energy requirements. As Glycolysis progresses, energy is gradually extracted for cellular metabolism, and Glycolysis produces a small amount of ATP.
Stochiometric Equation
Glucose + 2ATP +4ADP+ 2 NAD++ H3PO4 –> 2 Pyruvate + 2 ADP + 4 ATP + 2 NADH + H+ + H2O
In Glycolysis, energy is captured in the form of ATP and electron carrier NADH:
- For each glucose molecule processed through Glycolysis:
- 4 ATP molecules are produced
- 2 NADH molecules are produced
- However, 2 ATP molecules were invested in the preparatory phase.
- Therefore, the net ATP gain per Glucose molecule is 2.
- The generated NADH will continue to the electron transport chain, driving oxidative phosphorylation and more ATP production.
So, in summary:
Per Glucose Molecule
- Investment Phase: 2 ATP consumed
- Output Phase: 4 ATP produced
- Net Gain: 2 ATP and 2 NADH produced
Under anaerobic conditions, Glycolysis yields only 2 ATP per glucose molecule. However, subsequent aerobic respiration can yield up to 34 ATP with the completed oxidation of Glucose.
Fate of Pyruvate
The end product of Glycolysis is pyruvate. Under aerobic glycolysis conditions, pyruvate will continue to the next stage – the citric acid (TCA) cycle. Simply once the pyruvate
However, under anaerobic conditions, pyruvate’s fate depends on the organism:
1. Lactic Acid Fermentation
In muscles during exercise, pyruvate is converted to lactate by lactate dehydrogenase.
Pyruvate + NADH + H+ → Lactate + NAD+
It regenerates NAD+ needed for Glycolysis but causes fatigue due to lactic acid buildup.
2. Alcoholic Fermentation (Sugar fermentation)
In yeast, pyruvate is converted to ethanol and CO2 by pyruvate decarboxylase and alcohol dehydrogenase.
Pyruvate → CO2 + Acetaldehyde
Acetaldehyde + NADH + H+ → Ethanol + NAD+
This process is exploited for brewing beer, wine, and bread-making.
Regulation of Glycolysis
Glycolysis also serves as a link between other metabolic pathways, such as gluconeogenesis and the TCA cycle. As Glycolysis converts Glucose into intermediates, it is intertwined with these pathways, ensuring the cell’s overall metabolic balance and providing a crucial bridge for metabolites that enter the citric acid cycle. Thus, Glycolysis is an essential and intricate metabolic pathway with a range of enzymes involved, making it a fundamental part of cellular energy regulation.
Glycolysis is carefully regulated to meet cellular energy demands by three distinct mechanisms:
1. Allosteric Regulation
ATP and citrate allosterically inhibit the enzyme phosphofructokinase. When ATP is adequate, it slows down Glycolysis.
2. Substrate Availability
The availability of the substrate glucose controls the pace of Glycolysis.
3. Covalent Modification
Enzymes like phosphofructokinase can be regulated by phosphorylation/dephosphorylation of Ser/Thr residues.
4. Hormonal Regulation
- Insulin activates the key glycolytic enzymes – GlucoKinase (GK), Phospho Fructo Kinase (PFK) and Pyruvate Kinase (PK)
- Glucocorticoids inhibit Glycolysis.
These finely-tuned control mechanisms ensure glycolysis proceeds at the optimal rate to match energy needs at that time.
Glycolysis in Disease and Health
As the central pathway for glucose breakdown and ATP production, Glycolysis is indispensable in human health. However, dysregulation of this pathway is implicated in common diseases:
- Diabetes – Defects in glucose uptake due to insufficient insulin action lead to diabetes and metabolic disease.
- Cancer – Malignant cells exhibit an increased dependence on anaerobic Glycolysis for ATP generation, even in the presence of oxygen. It is known as the Warburg effect.
- Alcoholism – Alcoholic fermentation in humans contributes to ethanol toxicity and alcohol dependence.
A deeper understanding of the regulation and metabolism of Glycolysis will provide key insights into treating these prevalent modern diseases.
Frequently Asked Questions on Glycolysis
What is the primary purpose of Glycolysis?
The primary purpose of Glycolysis is to break down Glucose and harvest energy in the form of ATP and NADH. Glycolysis extracts only a small portion of the available energy from Glucose. But it primes Glucose for further breakdown through the citric acid cycle, which optimizes ATP production.
Where in the cell does Glycolysis occur?
Glycolysis occurs in the cytoplasm of both prokaryotic and eukaryotic cells, as none of its steps require membrane-bound organelles. Being in the cytoplasm allows Glycolysis to occur under aerobic and anaerobic conditions.
What is the rate-limiting step of Glycolysis?
The kinase enzyme phosphofructokinase is the rate-limiting and committed step of Glycolysis. It catalyzes the phosphorylation of fructose-6-phosphate to fructose-1,6-bisphosphate using another ATP molecule. This irreversible reaction prevents Glycolysis from reversing and pulls the pathway forward.
How is Glycolysis regulated in the cell?
Glycolysis is tightly regulated to meet cellular energy demands by three main mechanisms:
1. Allosteric regulation of phosphofructokinase by ATP and citrate.
2. Availability of the substrate glucose.
3. Covalent modification via phosphorylation/dephosphorylation.
Does Glycolysis require oxygen?
No, Glycolysis does NOT require oxygen. It can proceed under both aerobic and anaerobic conditions. It highlights Glycolysis as the most universal metabolic pathway that provides energy to cells even in low-oxygen environments. The presence or absence of oxygen determines the fate of pyruvate after Glycolysis.
Conclusion
Overall, Glycolysis converts one six-carbon molecule of Glucose into two three-carbon pyruvate molecules. Glycolysis is the essential starting point for glucose metabolism and energy generation in the cell. This conserved 10-step pathway manages the breakdown of Glucose to pyruvate with the production of ATP and NADH.
Understanding the intricacies of glycolysis regulation and integration with subsequent pathways has pivotal implications for human health and disease. In this guide, we have explored the steps, purpose, and key players involved in extracting energy from sugar through this fundamental process of Glycolysis.
Moreover, Glycolysis is involved in extracting energy and plays a pivotal role in linking with other metabolic pathways. It serves as a bridge between Glycolysis and gluconeogenesis, allowing for the conversion of intermediates back into Glucose when needed.
Additionally, Glycolysis by conversion ensures that some of its intermediates enter the TCA cycle, further contributing to energy production. This metabolic flexibility allows cells to maintain energy consumption under anaerobic conditions and precisely regulate Glycolysis, making it a central player in cellular energy homeostasis.