Intermediary Metabolism (Basic Notes)
One of the major reasons for studying Biochemistry is to understand how living organisms utilize the chemical energy in their environment to carry out their biochemical activities. It is called intermediary metabolism.
This requires an understanding of the simpler principles of physical chemistry and thermodynamics as they apply to live organisms.
Also required is an appreciation of the so-called “energy-rich compounds’ that permit the living organism to trap and subsequentially utilize the chemical energy contained in the food materials it consumes.
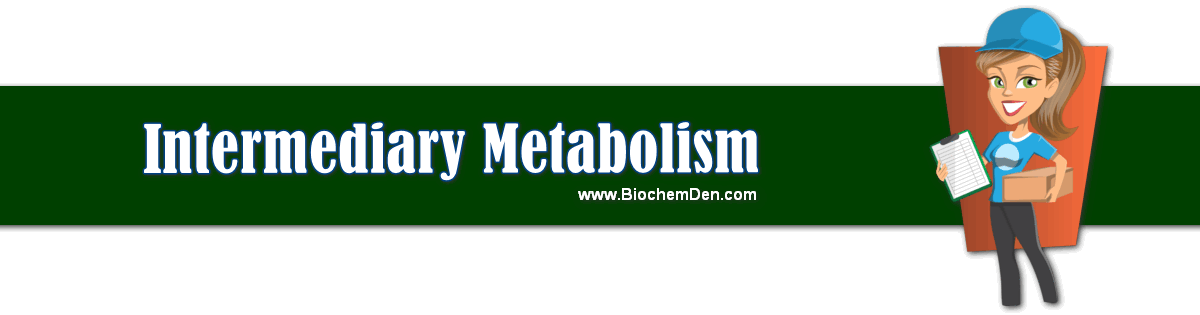
What is Metabolism?
The sun is the ultimate source of energy for all life on the planet Earth. That energy, like sunlight, is trapped by photosynthetic organisms and used to convert CO2 into the organisms’ cellular material, composed mainly of proteins, carbohydrates, and lipids, but also smaller amounts of nucleic acids, vitamins, coenzymes, and other compounds.
Some of those products of photosynthesis (carbohydrates and lipids) are, in turn, utilized by non-photosynthetic organisms, mainly animals, as a source of energy for growth, development, and reproduction.
Types of Metabolic Reactions
There are THREE types of reactions are present in Metabolism. They are
Exothermic Reactions
The reactions release energy and, therefore, have negative enthalpy changes. On reaction energy diagrams, the products of exothermic reactions have energy levels lower than those of starring materials.
Endothermic reactions
The reactions absorb energy and, therefore, have positive enthalpy changes. In reactions energy diagrammed, the products of endothermic reactions have higher energy levels than the starting materials.
Isothermic reaction
This type of reaction has not released any energy.
Bonds Cleavage and its types
In intermediary metabolism, a covalent bond (σ-bond) is formed with the help of two atoms by the sharing of a pair of electrons. When the two atoms are separated from each other, bond fusion (or cleavage) is said to have taken place.
The cleavage process can occur in two ways: a) homolytic cleavage and b) Heterolytic cleavage.
a) Homolytic cleavage
When one electron of the bonding pair goes with each of the departing atoms, the fission is symmetrical (0r) and homogenous, and is called “Homolytic cleavage” (or) “Homolysis”.
A-B (or) A:B –> A. + .B
- The two fragments that are produced as a result of hemolytic fission carry an odd electron each and are called “Free Radicals”.
- These are transitory and at once react with other radicals (or) molecules by giving one more electron to restore the stable bonding pair.
b) Heterolytic Cleavage
When a covalent bond breaks in a fashion that both the bonding electrons are appropriated by one of the two departing fragments (atoms or groups), it is said to have undergone “Heterolytic cleavage” (or) “Heterolysis“.
A-B (or) A:B –> A:– + B+
- The heterolytic cleavage yields one positive & one negative ion.
- These reactions take place more readily in polar solvents like water and are catalyzed by the presence of ionic catalysts (e.g.: acidic or basic).
Bond Length
Atoms involved in the formation of a bond cannot come any closer to each other than a certain distance where the potential energy is at its minimum.
Bond | Bond Length (A0) |
---|---|
C – C | 1.57 |
C = C | 1.34 |
C ≡ C | 1.20 |
C – N | 1.47 |
C = N | 1.28 |
C ≡ N | 1.15 |
C – O | 1.43 |
C = O | 1.20 |
C – H | 1.12 |
C – F | 1.42 |
C – Cl | 1.77 |
C – Br | 1.91 |
C – I | 2.13 |
O – H | 0.97 |
N – H | 1.03 |
S – H | 1.35 |
The average distance between the nuclei of two atoms bonded to each other is used under the name of bond length” or “bond distance.”
Thus, bond length may be defined as the average distance between the centers of the nuclei of the two bonded atoms. It is expressed in angstrom (A0) units (1A0 = 10-8 cm).
Points to remember:
- Multiple bonds (double or triple bonds between two atoms) are always shorter than the corresponding single bond.
- Bond length decreases with the increase in “s” character, since an s-orbital is smaller than a p-orbital. Thus
- sp3 C-H = 1.093 Ao (in alkanes)
- sp2C-H = 1.087 Ao (in Alkenes)
- sp C-H = 1.057 Ao (in Alkynes)
- Since bond distance is the sum of the ionic or covalent (atomic) radii of the two concerned atoms, the factors, and trends observed in the ionic or atomic radii will apply on the bond distance.
Bond Angle
In the molecules are made up of three or more atoms, the average angle between the bonded orbitals is known as a bond angle, Ф. Fundamentally, the value of the bond angle largely depends on the nature of the bonds concerned. The bond angle in the water molecule formed by the overlapping of two s-orbitals of hydrogen atoms with one 2p orbital of an oxygen atom should be 900. The actual H-O-H bond angle is 1040 31’. Bong angles are important for analyzing the behavior of molecules in intermediary metabolism.
Points to remember
- Repulsion between atoms or groups attached to the central atoms: the positive charge, developed due to the high electronegativity of oxygen, on the two hydrogen atoms in water causes repulsion among themselves, which increases the bond angle, H-O-H from 900 to 1050.
- Hybridization of bonding orbitals: hybridization of bonding orbitals also plays a very important role in determining the values of bond angles.
Bond type | sp3 | sp2 | sp |
Bond angle | 1090 28’ | 1200 | 1800 |
- Repulsion due to non-bonded electrons: the deviation of the normal tetrahedral bond angle of 1090 28’ in H2O (1050), NH3 (1070), etc. although their central atoms are in an sp3 hybridized state.
Classification of Metabolic Reagents
Now let us discuss the metabolic reagents in intermediary metabolism. The presence of a charge on the reactant certainly helps the attack of the reagent on the reactant, but it is far from essential.
Indeed, the requisite unsymmetrical charge distribution may be induced by the mutual polarization of the reagent and reactant on their close approach, as when bromine is added to ethylene.
Thus, because of the above principle, most of the reagents can be classified into the following two types.
1.Electrophilic reagents (Electrophiles)
The name implies electrophilic (electro=electron, phile=love) reagents are electron seeking (or) loving and thus attack the substrate at the point of maximum electron density. Thus, an electrophile is a species having an electron-deficiency atom (or) center.
The electrophilic reagent may be a positively charged species or a neutral molecule with an electron-deficient center. Some important electrophiles are given below:
E.g.: H+, Br+, NH4+, SO3, R-N+≡N,
It is interesting to note that since the electrophiles are capable of accepting electrons pair, they are Lewis acids.
Reactions involving the attack of electrophiles are known as an electrophilic reaction.
2. Nucleophilic reagents (Nucleophiles)
The reagent possessing at least one lone pair of electrons is known as nucleophilic reagents (or) nucleophiles (nucleo=nucleus; phile=love).
Since they possess a higher electron density, they attack the substance at the point of minimum electron density. The nucleophilic reagent may be a negatively charged species or neutral molecule with free electron pair (s).
Some important nucleophiles are given below:
E.g.: OH–, Br–, CN–, C00–, R.C≡C–, CH3COC–H2, H-O-H, R-O-R, R-O-H, R-S-H, R-NH2, R2-NH2, :NH3, LiAlH4.
It is interesting to note that since the nucleophiles are capable of donating electrons pair, they are Lewis bases.
The reaction involving the attack of nucleophiles is referred to as a nucleophilic reaction.
Metabolic Phases
Metabolism refers to the biochemical processes that occur within living organisms to maintain life. It involves the conversion of molecules (metabolites) through various chemical reactions to produce energy, synthesize biomolecules, and carry out other essential cellular functions.
Thousands of chemical reactions are taking place inside a cell in an organized, well co-organized, and purposeful manner; all these are collectively called Intermediary Metabolism. The term Metabolism came from the Greek language (metaboleG = Change; balleinG= to throw).
In living organisms, there are three different phases of metabolic phases done. These are :
- Primary Metabolism: The primary metabolic stateincludes digestion.
- Secondary Metabolism: The secondary metabolism is also called the intermediary metabolism. It includes catabolism (breakdown process of biomolecules) and anabolism. (Synthesis process ofbiomolecules).
- Tertiary Metabolism: Biological oxidation and Oxidative phosphorylation are included in this type of metabolism.
Intermediary metabolism serves the following purposes
Metabolism is the overall process through which living systems acquire and utilize the free energy they need to carry out their functions. The terms “catabolism” and “anabolism” were coined by the physiologist Gasket in 1886.
- Chemical energy is obtained from the degradation of energy-rich nutrients.
- Food materials are converted into the building block precursors of cellular macromolecules. These building blocks are later made into macromolecules, such as proteins, nucleic acids, polysaccharides, etc.
- Biomolecules required for specialized functions of the cell are synthesized. Specialized functions of the cell are synthesized.
- Metabolic pathways are taking place with the help of sequential enzyme systems. These pathways are regulated at three levels:
- Regulation through the action of allosteric enzymes, which increases or decreases in the activity under the of effector molecules.
- Hormonal regulation: Hormones are chemical messengers secreted by different endocrine glands.
- Regulation at the DNA level: The concentration of the enzyme is changed by regulation at the level of synthesis of the enzyme.
Metabolism can be broadly categorized into two types of metabolic reactions: catabolic and anabolic.
a) Catabolism
It is a degradation process. A degradative process in which complex molecules are broken down into simpler ones; includes processes such as Cellular Respiration and digestion. The meaning of catabolism came from Greek (kataboleG = Throwing down; balleinG= to throw). It is also spelled as katabolism. The energy released during this process is trapped chemical energy, usually ATP. Eg: Cellular Respiration
The primary purpose of catabolism is to generate energy and provide building blocks for anabolic reactions. Examples of catabolic reactions include:
- Glycolysis: Breaking down glucose into pyruvate, producing ATP and NADH.
- Krebs cycle (TCA cycle): The oxidation of acetyl-CoA derived from glucose, fatty acids, or amino acids to produce ATP, NADH, FADH2, and carbon dioxide.
- Beta-oxidation: The breakdown of fatty acids into acetyl-CoA, generating NADH and FADH2.
- Protein degradation: The breakdown of proteins into amino acids, which can be used for energy production or other cellular processes.
- Fermentation: The anaerobic breakdown of glucose or other organic compounds to produce energy in the absence of oxygen.
b) Anabolism
Anabolic reactions involve synthesizing complex molecules from simpler molecules, consuming energy. These reactions build cellular structures, store energy, and produce biomolecules necessary for cellular function. It is a biosynthesis process. A constructive process in which complex molecules are synthesized from simpler ones; consumes rather than produces cellular energy; includes processes such as photosynthesis and assimilation; the opposite of catabolism. The meaning of the word “anabolism” comes from the Greek (AnaboleG = Throwing up; balleinG= to throw). This needs energy. Eg: Photosynthesis
Examples of anabolic reactions include:
- Protein synthesis is assembling amino acids into polypeptide chains to form proteins.
- Lipogenesis: The synthesis of fatty acids and triglycerides from acetyl-CoA and other precursors.
- Glycogenesis: The synthesis of glycogen from glucose molecules for storage.
- Nucleotide synthesis: The production of nucleotides, the building blocks of DNA and RNA.
- Photosynthesis: The process by which plants and some microorganisms convert sunlight, carbon dioxide, and water into glucose and oxygen.
These metabolic reactions, catabolism and anabolism, are interconnected and work harmoniously to balance energy and molecular components within cells.
Catabolic reactions provide the necessary energy and building blocks for anabolic reactions, while anabolic reactions utilize the energy and building blocks to construct complex molecules essential for cellular processes. Coordinating these reactions is critical for living organisms’ proper functioning and survival.
Metabolic Pathways
Metabolic pathways are a series of interconnected chemical reactions within cells to convert one molecule into another, ultimately leading to the synthesis or breakdown of various molecules. These pathways are essential for the proper functioning of cells and play a crucial role in energy production, nutrient metabolism, and the synthesis of biomolecules.
An array of enzyme-catalyzed chemical reactions that bring about transformations of certain organic compounds vital to the organism, constitute “Metabolic pathways” (or) “Metabolic routes”.
Here are some key metabolic pathways:
Glycolysis:
- Glycolysis is the pathway by which glucose is broken down into pyruvate.
- It occurs in the cytoplasm and produces a small amount of ATP and NADH.
Citric Acid Cycle (TCA Cycle):
- Also known as the Krebs or tricarboxylic acid cycle, it occurs in the mitochondria.
- Acetyl-CoA, derived from glucose, fatty acids, or amino acids, enters the cycle and is oxidized to produce NADH, FADH2, and ATP.
Oxidative Phosphorylation:
- This pathway takes place in the inner mitochondrial membrane.
- It involves the electron transport chain, where electrons from NADH and FADH2 are passed along a series of proteins, creating a proton gradient.
- The flow of protons back across the membrane generates ATP through ATP synthase.
Beta-Oxidation:
- Beta-oxidation is the process by which fatty acids are broken down into acetyl-CoA.
- It occurs in the mitochondria and generates NADH and FADH2, which enter the TCA cycle.
Pentose Phosphate Pathway:
- The pentose phosphate pathway operates parallel to glycolysis.
- It produces NADPH and generates intermediates for the synthesis of nucleotides.
- Gluconeogenesis is the reverse process of glycolysis, occurring primarily in the liver.
- It converts non-carbohydrate precursors (lactate, amino acids, and glycerol) into glucose.
Urea Cycle:
- The urea cycle occurs in the liver and converts toxic ammonia into urea for excretion.
- It involves a series of reactions that occur between the mitochondria and cytoplasm.
Protein Synthesis:
- Protein synthesis involves the transcription of DNA into messenger RNA (mRNA) and the subsequent translation of mRNA into proteins.
- It occurs in the nucleus (transcription) and cytoplasm (translation) and requires amino acids, tRNA, ribosomes, and other factors.
These are just a few examples of metabolic pathways, and many more are involved in various other processes, including lipid synthesis, amino acid metabolism, and nucleotide synthesis. The regulation and coordination of these pathways ensure the efficient utilization of nutrients and energy to meet the needs of cells and organisms.
Three Principle characteristics of metabolic pathways, there are Irreversible pathways, Typical irreversible metabolic pathways, and Branched metabolic pathways.
1. Irreversible pathways
These are highly exergonic reactions. If two metabolites are metabolically inter-convertible, the pathway from the first to the second must differ from the pathway from the second back to the first. Example of irreversible pathways is Carbohydrate Oxidation, Fatty acid Oxidation, and Heme Biosynthesis.
2. Typical irreversible metabolic pathways
To obtain the product, a metabolic sequence should be essentially irreversible. Examples fro typical metabolic pathways are Glycolysis, Synthesis and Degradation of liver glycogen from (or) to Glucose.
3. Branched metabolic pathways
Some metabolic sequences may have a common path for many steps and then branch into two (or) more separate paths. Examples to the Branched metabolic pathways are, in carbohydrates metabolism, “Glucose-6-Phosphate” act as starting precursor molecule for the following pathways. Glycogen synthesis, EMP pathway (or) Glycolysis, HMP shunt.
What are the Principle characteristics of metabolic pathways?
The principal characteristics of metabolic pathways include:
- Interconnectedness: Metabolic pathways are interconnected networks of chemical reactions. The products of one reaction serve as substrates for subsequent reactions, creating a chain of interlinked steps. It allows for the efficient utilization of resources and coordination of metabolic processes.
- Irreversibility: Metabolic pathways are often irreversible, meaning the reactions proceed in one direction. While some reversible reactions do occur, the overall flow of the path is typically unidirectional, driven by energy considerations and regulatory mechanisms.
- Regulation: Metabolic pathways are tightly regulated to ensure proper control of metabolic flux and respond to changing physiological conditions. Regulation can occur at various levels, including enzyme activity, substrate availability, and gene expression. This regulation allows cells and organisms to adapt to energy demands and nutrient availability.
- Energy Coupling: Metabolic pathways are energetically coupled, meaning energy released in one reaction is often used to drive an unfavourable reaction. For example, glycolysis energy generated during glucose oxidation is used to synthesize ATP through oxidative phosphorylation.
- Catabolic and Anabolic Pathways: Metabolic pathways can be categorized as catabolic or anabolic. Catabolic pathways involve breaking complex molecules, releasing energy and producing simpler molecules. Anabolic pathways, on the other hand, involve the synthesis of complex molecules, requiring energy input and building blocks.
- Compartmentalization: Metabolic pathways are often compartmentalized within specific organelles or subcellular structures. This compartmentalization allows for the spatial organization and separation of incompatible reactions. For example, glycolysis occurs in the cytoplasm, while oxidative phosphorylation occurs in the mitochondria.
- Feedback Inhibition: Many metabolic pathways are regulated by feedback inhibition. It occurs when the final product of a pathway acts as an inhibitor of an earlier enzyme in the pathway, regulating the rate of the pathway and preventing the over-accumulation of end products.
- Diversity: Metabolic pathways exhibit tremendous diversity across different organisms and cell types. While core metabolic pathways are conserved, variations and adaptations exist to meet the specific metabolic needs of other organisms and their environments.
These characteristics collectively contribute to the efficiency, adaptability, and regulation of metabolic pathways, ensuring the proper utilization of nutrients, energy production, and maintenance of cellular homeostasis.
Intermediary Metabolism
Metabolism is the term used to describe the interconversion of chemical compounds in the body, the pathways taken by individual molecules, their interrelationships and the mechanisms that regulate the flow of metabolites through the pathways.
Intermediary metabolism refers to the network of biochemical reactions within cells that process and convert nutrients into energy, building blocks for cellular structures, and other molecules necessary for cellular function. It involves the metabolism of carbohydrates, lipids, and proteins. Here are the key aspects of intermediary metabolism:
1. Carbohydrate Metabolism
- Glycolysis: Carbohydrates, mainly glucose, are broken down into pyruvate through a series of enzymatic reactions in the cytoplasm. This process generates a small amount of ATP and NADH.
- Pyruvate Decarboxylation: In the presence of oxygen, pyruvate enters the mitochondria and undergoes decarboxylation to form acetyl-CoA, which enters the tricarboxylic acid (TCA) cycle.
- Tricarboxylic Acid (TCA) Cycle: Also known as the citric acid cycle or Krebs cycle, the TCA cycle oxidizes acetyl-CoA and produces energy-rich molecules such as NADH and FADH2.
- Oxidative Phosphorylation: NADH and FADH2 are generated during glycolysis, pyruvate decarboxylation, and the TCA cycle donates electrons to the electron transport chain in the mitochondria. This process leads to the production of ATP through oxidative phosphorylation.
2. Lipid Metabolism
- Lipolysis: Triglycerides stored in adipose tissue are broken down into glycerol and fatty acids through the action of lipases.
- β-Oxidation: Fatty acids are transported into the mitochondria and undergo a series of reactions called β-oxidation, producing acetyl-CoA, NADH, and FADH2.
- Ketogenesis: Under conditions of prolonged fasting or low carbohydrate intake, excess acetyl-CoA generated from fatty acid breakdown enters ketogenesis, leading to the production of ketone bodies (acetoacetate, β-hydroxybutyrate, and acetone).
- Lipogenesis: In the fed state, when energy is abundant, excess glucose and acetyl-CoA are converted into triglycerides through lipogenesis.
3. Protein Metabolism
- Protein Digestion: Dietary proteins are broken down into amino acids through digestion in the stomach and small intestine.
- Protein Synthesis: Amino acids synthesize new proteins necessary for cellular structure, enzymes, hormones, and other functional molecules.
- Amino Acid Metabolism: Amino acids can also be converted into intermediates for energy production or used to synthesize non-protein molecules, such as neurotransmitters, nucleotides, and heme.
These metabolic pathways are highly interconnected, allowing for the efficient utilization of different nutrients depending on the energy demands and availability of substrates. Intermediary metabolism plays a vital role in maintaining energy balance, providing building blocks for cellular components, and regulating various physiological processes in the body.
Pathways that process the Major Products of Digestion
The nature of the diet sets the basic pattern of metabolism. There is a need to process the products of digestion of dietary carbohydrates, lipids, and protein.
These are mainly glucose, fatty acids and glycerol, and amino acids, respectively. All the products o digestion are metabolized to a common product, acetyl~coA, which is then oxidized by the citric acid cycle.
Metabolism can be considered in different types based on the Biomolecule:
- Carbohydrate Metabolism
- Amino acid Metabolism
- Lipids Metabolism
- Nucleotide Metabolism
- Porphyrin Metabolism
- Vitamin Metabolism
- Mineral Metabolism